Scientists Used Neutrinos to Map the Milky Way. It Could Help Them Find Dark Matter and Gravitational Waves
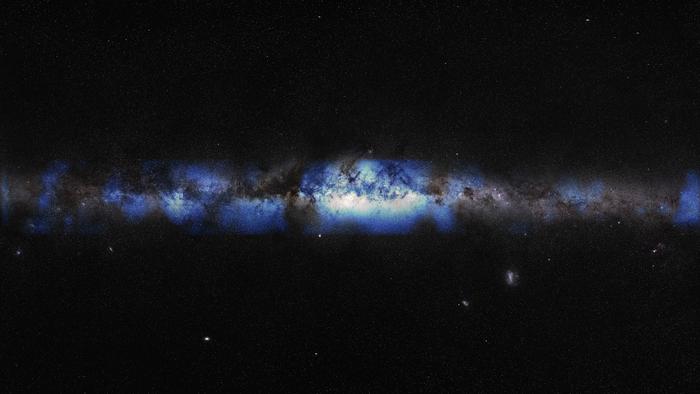
One of the most ghostly forms of radiation, neutrinos are notoriously difficult to detect. Produced in the Sun and in deep space, tens of billions of the chargeless, nearly-massless particles pass through every square centimeter of Earth every second, breezing through most matter and magnetic fields with barely a whisper of interaction. Even the one-cubic-kilometer–sized IceCube Neutrino Observatory, buried 2,500 meters beneath the Antarctic snow to shield it from interfering forms of radiation, has trouble pulling signs of neutrinos out from the background noise of cosmic rays and high energy particles generated in Earth’s own atmosphere. And yet, a massive international team of scientists has recently developed a way to use these elusive neutrinos to draw up new maps of the Milky Way.
“Even with our large detector,” says University of Maryland Particle Physicist Erik Blaufuss. “We’re still only seeing hundreds” of neutrinos coming from the Milky Way galaxy — following a 10-year search.
Given their subtle nature, one wouldn’t then expect neutrinos to function as the basis of a new type of astronomical observation, but some of the recent findings of the IceCube Collaboration, an international team of more than 300 scientists (including Blaufuss), suggest exactly that. In a recent paper published in the journal Science, he and his colleagues created a map of neutrino “light” across our galaxy. Those maps can then in turn be used to help investigate other mysterious phenomena, such as dark matter, gravitational waves, and the unknown sources of gamma radiation and cosmic rays coming from areas of the inner core of our galaxy.
“Neutrinos have the additional power that they can see into sources that the gamma rays can’t,” Blaufuss told SpaceRef. Dense environments like the galactic core are difficult to map using gamma rays, because they will be absorbed and shifted to lower wavelengths of electromagnetic energy by the material around them. Meanwhile, Blaufuss said, “That material is not enough to stop the neutrinos.”
Neutrinos are, of course, a subject of scientific interest in their own right. They’re produced by radioactive decay — even the decay of potassium in bananas emits neutrinos — and in nuclear fission and fusion reactions, meaning the sun is a major local source of neutrinos.
But neutrinos are also produced in deep space when galactic cosmic rays, high-energy protons accelerated to immense velocities by unknown sources, interact with interstellar gas, producing both gamma rays and, scientists had theorized, high-energy, “cosmic” neutrinos.
“If you’re seeing gamma rays from these interactions,” Blaufuss said, “it’s a strong expectation that neutrinos would be there.”
The new study, which combined clever observational and data analysis techniques to highlight cosmic neutrino sources within the Milky Way, confirmed that hypothesis.
The IceCube Observatory relies on 80 optical sensors designed to catch the flash of blue light created during an “event,” or the rare occasion a neutrino impacts a proton inside the detector. But neutrinos are not the only thing that can trigger the sensors, according to Blaufuss. Despite being buried deep beneath the ice, charged particles created by cosmic rays striking atoms in Earth’s atmosphere also pass through IceCube.
And even when it is a neutrino that triggers an IceCube sensor, it’s not always clear whether it came from a galactic source or the Sun. Blaufuss explained that the IceCube team uses a technique called “event stacking,” which involves identifying neutrino signals that line up with known positions of gamma ray sources in the galactic plane, for instance, to help distinguish cosmic neutrinos from solar neutrinos or other forms of radiation hitting the IceCube sensors.
“Using machine learning techniques to reconstruct their direction and make their selection more efficient, we were able to get a lot more signal neutrinos from the galaxy,” he said, “and pull that image out of the background.”
Now that the IceCube team has demonstrated its ability to identify neutrino signals, it can map those signals onto other astronomical signals and processes to better understand them. For instance, IceCube could help pinpoint the exact location of the source of the gravitational waves — ripples in spacetime itself generated by the mergers of massive neutron stars — currently being hunted by the Laser Interferometer Gravitational-Wave Observatory (LIGO).
“It takes another measurement to be able to pinpoint the actual location of the gravitational merger that created that gravitational wave,” Blaufuss said. “We’re trying to use neutrinos to do that. Other people are using photons.”
And because cosmic neutrinos originate from cosmic ray interactions, neutrino astronomy may in turn help astronomers pinpoint the mysterious origins of these high-energy rays zipping through the galaxy.
“Something out there has to be accelerating these particles to these phenomenal energies that we see here on Earth,” Blaufuss said, “that are 10 orders of magnitude larger than anything we can do on a terrestrial scale in terms of [the Large Hadron Collider].”
Perhaps most intriguing of all, neutrinos may provide clues to the nature of dark matter, the enigmatic material that, despite the fact that it’s pervading the universe, is so far only indirectly visible to scientists who must measure the effects of its mass on ordinary matter.
One hypothesis is that “dark matter will annihilate with itself and make a spew of particles, including neutrinos,” Blaufuss says. ”We can use neutrinos as a signature for dark matter,” at places where it’s theorized to clump together, including the centers of our galaxy, the Sun, and even Earth itself.
There are hard limits to neutrino astronomy, however, primarily that the rate of detection of neutrinos at individual sensors is already as good as it can ever get. The flux, or the amount of neutrinos passing through an area each second, is set by nature, Blaufuss explained, as is the likelihood that any given neutrino will interact with other matter. So to take things to the next level you need a bigger neutrino observatory.
“That’s a slower time scale thing because we’re already a cubic kilometer detector,” he said. “So the next kind of scale would be a 10 cubic kilometer detector, which will take a bit of money and time to develop it. Because it’s built into the South Pole, so you can really only work during the summer down there.”