Modern Analysis Of Apollo Moon Rocks
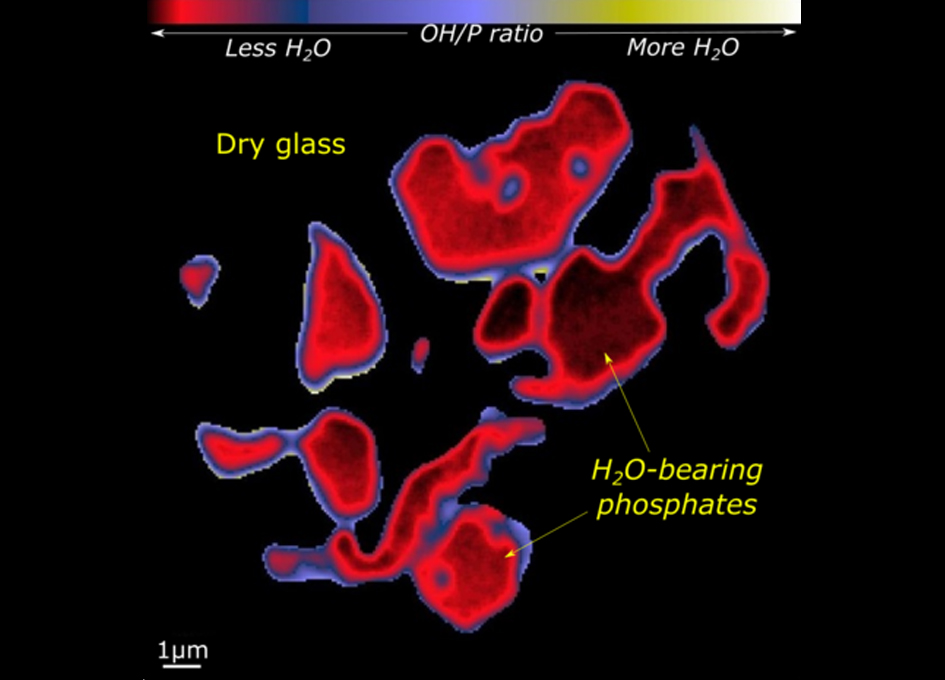
On July 20, 1969, as Apollo astronaut Neil Armstrong climbed down the ladder from the “Eagle” lunar landing module, he found himself surrounded by a sea of grey — an expanse of powdery dust no human had ever seen in person.
The iconic print made by his left boot marked but the first step on a long journey of discoveries about the Moon and our own world — both of which hold secrets that scientists are only beginning to uncover.
Fifty years after the Apollo astronauts collected samples of Moon rocks and dust during their forays across the lunar landscape, there are still mysteries to be solved, and one University of Arizona scientist is looking for answers. Jessica Barnes, an incoming assistant professor in the UA’s Lunar and Planetary Laboratory, was recently selected by NASA to receive access to preciously unopened Moon rock samples.
Under NASA’s Apollo Next Generation Sample Analysis, or ANGSA, program, Barnes will be granted access to Apollo 17 sample 71036, which contains almost four ounces of rock. Several samples from that mission were initially processed under nominal laboratory conditions, protected from air exposure by a nitrogen cabinet at room temperature, and placed into cold storage within one month of return.
“When these samples were brought back, the curators had the foresight to say, ‘In this moment, we don’t have all the methods to answer all the questions these samples could help us answer,’ and so they locked some away for future study,” Barnes says. “They realized that future technologies would allow us to do things that would have been impossible at the time, and that people would come up with new questions. It’s really exciting because we’re at that point in time now.”
Barnes is on a quest to find out where water came from in the early solar system and how it has evolved over time. Previous research, including some of her own work, suggests that certain space rocks known as carbonaceous chondrites brought water with them when they impacted Earth and Mars, and potentially some of the larger asteroids. It is no coincidence that Bennu, the target asteroid of the UA-led OSIRIS-REx sample return mission, is a carbonaceous chondrite.
“To understand where water in the solar system came from, and particularly how it ended up on Earth, Mars, and in the asteroid belt, we have to consider the Moon,” says Barnes, whose current research focuses on tracing water meteorites, including some of Martian origin, and Moon samples collected during Apollo 11, 14, and 17. “Understanding how life on Earth began is intimately tied to the story of how water arrived here. Lunar samples are critical pieces in this puzzle because unlike Earth, where the oldest rocks have largely been erased by plate tectonics, the Moon’s ancient rock record is still intact.”
About 4.6 billion years ago, when a swirling nebula of gas and dust began collapsing into a disk that would give rise to our solar system, the rocky planets and the carbonaceous chondrites were developing in different places and at different times, Barnes explains, which poses a problem for the scenario involving early asteroids as harbingers of water.
“It was only 10 years ago that water was discovered on the Moon, not only on the surface, but also inside minerals,” Barnes says. “In science, that is a pretty short timescale, and we don’t have it all figured out yet. How much water is there? Did it come from the Earth during the great impact that we think created the Moon, or was it given to the Moon later? Is it distributed uniformly or in patches within the Moon’s mantle?”
To find answers to such questions, Barnes, who wasn’t even born when the Apollo astronauts crisscrossed the lunar surface on foot and with their rovers, is using technology that wasn’t invented until the early 2000s.
Ion Beams And Atom Smashers Expose Secrets of Moon Rocks
“When you first receive your sample, you don’t know what you are looking at, so you start with a visual analysis,” Tom Zega says, pointing to a simple dissecting microscope, like the ones used in introductory science labs. Zega is an associate professor of planetary sciences, and materials science and engineering, and co-investigator on the ANGSA project. He also is director of the Kuiper Materials Imaging and Characterization Facility at the LPL, a state-of-the-art facility designed with one goal: extract as much information as possible from samples, both terrestrial and extraterrestrial.
Studying a piece of Moon rock under an optical microscope is only the first step in a series of analytical techniques UA researchers have at their disposal. At the end is a 12-foot-tall transmission electron microscope, or TEM. Funded by the National Science Foundation and NASA, its serial number is “1” because it is the first of its kind in the world with this exact configuration. Its 200,000-volt electron beam can probe matter down to 78 picometers, scales too small for the human brain to comprehend.
“If you want to know what an atom from the birth of our solar system looks like, I can show you,” Zega says. To get a sample to where it gives up that much detail of its origin and history, however, requires a suite of complex instruments and expertise that no single discipline can provide.
“Today, all the interesting science happens at the intersection of various fields,” Zega added. “In my group, we have cosmochemists, quantum chemists, astrophysicists and astrodynamics, among others. This work requires a unique mix of knowledge and skills. Take the TEM, for example: it’s a quantum-mechanical tool, so you have to be an expert in physics, materials science and chemistry, all at the same time.”
Another instrument, called an electron microprobe, allows researchers to discover certain properties of a sample by scanning it with an electron beam. As it does, a spatial image of the sample emerges, in this case revealing an abstract, speckled landscape of light and dark areas that cosmochemists can read like a map.
“Heavier elements appear brighter, and lighter elements appear darker,” Zega says. “So this tells us, for example, where and how much iron there is compared to oxygen in a lunar sample.”
When Barnes moves to the UA this fall, after wrapping up her current research at NASA’s Johnson Space Center, she hopes to be able to expand the capacities of the Kuiper Materials Imaging and Characterization Facility with a next-generation NanoSIMS instrument, which stands for nanoscale secondary ion mass spectrometry.
The beauty of this technology, she says, lies in its ability to analyze isotopes, essentially different “varieties” of chemical elements, at very small scales — less than one-fiftieth the width of a human hair. Measuring the composition of different volatile elements such as hydrogen and chlorine in the rock tells the researcher something about the chemical make-up of the magma from which the rock crystallized and how its chemistry evolved over time.
“These data allow us to understand the chemistry of the Moon’s interior,” Barnes says. “Ultimately we are able to say something about how the Moon evolved and where its water came from.”
The possibilities don’t end here. To a curator during the Apollo days, a focused ion-beam scanning electron microscope, or FIB-SEM, would have sounded like utter science fiction: By smashing the bonds between atoms inside the sample with a beam of heavy gallium ions, the instrument works essentially like a nano scale excavator, Zega explains.
“Except that compared to other FIBs, which act like shovels, this one is a scalpel,” he says.
FIB-SEM allows scientists to cut out tiny pieces from a sample with high precision and analyze only those pieces. This technique recently enabled Zega’s team to discover a grain of dust forged in the death throes of a star long before our solar system was born.
“What we want to know from our samples is, how well do they conform to how we think the solar system formed based on astrophysical models?” Zega says.
The same applies to the origin of the Moon, Barnes says.
“It’s not just analytical instruments that have improved. In the last 10 years, major advancements in impact simulations and numerical modeling have allowed the community to simulate the speed, size and number of the bodies that might have been involved in creating the Earth-Moon system.”
Analyzing samples from extraterrestrial bodies goes beyond the origins of the Earth and the Moon, of course. They are critical pieces in the puzzle because they allow scientists to test hypotheses about formation processes in the solar system based on simulations and models.
“We have had lunar samples here for decades,” says Timothy Swindle, director of the LPL. “Our faculty have been studying the composition of the Moon for a long time, and what’s so special about these samples is that they were valuable 50 years ago, and they will be valuable 50 years from now.”
“Being able to study these previously unopened samples is like a whole new lunar sample return mission,” Barnes says of the value of studying 50-year-old Apollo samples. “Not only do we get to be a part of the history of opening these samples, but we also will be using this opportunity to study how curation practices, such as ambient versus cold storage, affect our ability to measure a lunar water signature.
“It’s exciting because this has never been done before.”
The University of Arizona, a land-grant university with two independently accredited medical schools, is one of the nation’s top 50 public universities, according to U.S. News & World Report. Established in 1885, the UA is widely recognized as a student-centric university and has been designated as a Hispanic Serving Institution by the U.S. Department of Education. The UA ranked in the top 25 in 2018 in research expenditures among all public universities, according to the National Science Foundation, and is a leading Research 1 institution with $687 million in annual research expenditures. The UA advances the frontiers of interdisciplinary scholarship and entrepreneurial partnerships as a member of the Association of American Universities, the 62 leading public and private research universities in the U.S. It benefits the state with an estimated economic impact of $4.1 billion annually.