Using a Planetary Analog To Test a Prototype Inflated Habitat for NASA
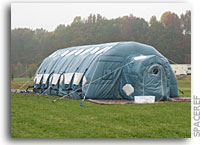
I had a chance to visit the manufacturing facilities at ILC Dover in Frederica, Delaware last week to see the new inflatable habitat that they have developed. Together, NASA, ILC Dover, and NSF will put this habitat through a one-year test at McMurdo Station in Antarctica starting in January 2008.
How this team came to chose this design – and develop it to this point of development – reaches back decades for its inspiration – also uses cutting edge technology to bring it to reality.
Inflatable technology
When you look at artist’s impressions of what a Moon base (or a Mars base) might look like, you usually see a bunch of cylinders. The cylinders are either lying on their side or standing upright and are made of metal with rocket engines underneath. Indeed, most of the new proposals NASA tosses around for the base it plans to build at the Moon’s south pole, feature variations on this same approach.
There is another approach to take, however. And it is not exactly a new one either. This other approach involves the use of inflatable, expandable structures. While some Apollo era space architects suggested inflatable structures, the technology had yet to mature. Flash forward 40 years and structures – some of them exceptionally large (Denver’s airport) are built with flexible fabrics.
In the 1990s NASA embraced inflatable designs in a big way. Called “Transhab“, NASA JSC put forth a design for a module that would hang off of the International Space Station and serve as a pathfinder for more advanced structures to be used on spacecraft and planetary surfaces. Alas, JSC was toying with human missions beyond low Earth orbit at a time when the White House forbid such notions. Transhab activity was shut down and the technology was put in a box.
Eventually, millionaire Robert Bigelow bought much of the intellectual property associated with Transhab. Adding in some of his own technology Bigelow eventually launched two inflatable spacecraft – with plans for an inhabited, commercially operated, space station to follow.
Advantages
Without getting into a long engineering treatise, inflatable structures offer a lot of desirable characteristics – in space – and on a planet’s surface. The one obvious advantage is ease of transport and set up. I speak from a certain amount of experience in this regard having spent time at the Haughton Mars Project Research Station on Devon Island on three occasions (2002, 2003, 2007). On my first trip I managed the construction of a 12×24 foot greenhouse (the Arthur Clarke Mars Greenhouse) that my company, SpaceRef Interactive, donated to the project.
Despite having done a test assembly of the greenhouse inside Hangar 1 at NASA Ames Research Center, actually building it – often times during cold, howling near-blizzard conditions – was another matter all together. All told it took more than a week to get all the pieces put together. A lot of planning went into this and we pulled it off more or less as planned. However, were I to do it again, I would do many things much differently- based on the experience I gained from actually building it on Devon Island.
I also helped erect several of the large quasi-permanent tents that comprise the core infrastructure of the research station. Each one is comprised of a steel frame that is assembled piece by piece. Even with a half a dozen people dedicated to the task it can take hours to put one up – and that assumes that there is already a wooden platform constructed underneath.
As I watched at the ILC Facility, the inflatable hab went from a flat pile of fabric to a fully erected, tied down, and ready to use structure in 11 minutes. If only we could set things up that fast on Devon Island! As such, one indisputable aspect of an inflatable’s utility is speed of installation. If you are going to send things to the moon or Mars ahead of human crews, then such ease of installation becomes abundantly apparent.
There is also the issue of weight and volume. Clearly if you can reduce weight and volume as you seek to send payloads to a remote location, you are doing yourself a big favor. Inflatable structures also offer that.
As to issues associated with radiation shielding, UV breakdown, temperature ranges, and other factors that become important on other worlds, inflatable structures offer benefits there as well. However, on the Moon and Mars these materials will need to be much more resilient, robust, and durable than things used on Earth today. But how we use those inflatable structures on other worlds is something we can start to learn about right now.
The Team
This habitat came about via an unusual pairing of government and the private sector. Specifically between NASA, NSF, and ILC Dover. NASA’s Innovative Partnership Program signed a Cooperative Agreement with ILC. NASA invested $295,000 in the project, as did ILC Dover. The habitat module was then designed and built by ILC Dover. The company was a natural for such an endeavor given that it has decades of experience – not only with spacesuits, but also with a variety of inflatable and fabric structures.
NASA then turned to NSF to set up the means where by the hab module would be delivered, set up, operated, taken down, and return from Antarctica – specifically McMurdo Station. This cooperation between NASA and NSF was coordinated via the Space Act Agreement that is in place between NASA and NSF. NASA and ILC are responsible for al the expenses of getting the hab and personnel to a staging location in New Zealand. From that point NSF takes over and covers the expenses. This is actually a standard process with regard to how much U.S. research is supported in Antarctica.
The Benefits
NASA and ILC gain the benefit of trying out the operations of an inflatable structure in a remote hostile location. The materials used to build the habitat – as was the case with our greenhouse on Devon Island – are materials for use on Earth – not on another world. That is not the point i.e. this is not a lunar material test run. The only pace to try that on Earth would be in a vacuum chamber. What is being examined is what it takes to deploy and then run something like this in one of the nastiest places you can find.
NSF gets something out of this as well. In addition to its own support of scientific research in space and other areas, it has a logistical and operational issue to deal with every day in Antarctica. While McMurdo Station and other major bases are comprised mostly of expensive, sturdy long-term structures, much of what is done in Antarctica is done with temporary structures that need to be set up, used, and then taken down for use elsewhere – on a project-by-project or seasonal basis. The workhorse for such structures is called the “Jamesway”.
In use since the 1950s, a Jamesway is a long, cylindrical structure – something that looks like a World War II era Quonset hut. The Jamesway is comprised of a metal skeleton overlaid with several layers of canvas and filled with fiberglass insulation. While this basic design has a half-century track record, it is cumbersome to erect and has other limitations as well. Having a structure that can be established in minutes would have a clear advantage to the NSF. Doing this field test in Antarctica where the Jamesway has been somewhat of a standard approach allows a very good field evaluation to be done of how the inflatable habitat performs in locations where Jamesways are experiencing the same operational conditions.
Of course, if you have limited resources on a place such as the Moon and Mars and you are traveling from one location to another, it would certainly be advantageous to carry as much habitable space as possible with a minimum amount of assembly and disassembly.
As such NASA, ILC, and NSF all have individual and common benefits they expect derive from this project.
The Hab Structure
You enter the habitat through an airlock that is 6 feet wide and 4 feet long (24 square feet of floor space) with a ceiling that is 6.5 feet high. The inside of the habitat itself has 384 square feet of floor space covering an area 24 feet long and 16 feet wide. The ceiling is 8 feet high but you can stand right up against the wall and still have ample clearance for the top of your head. When it is deflated and stowed for shipping, the habitat and its insulation weighs 1,100 pounds and fits into two 4′ by 4′ by 8′ packages (128 cubic feet each). The airlock compresses into a separate package that is the same size and weighs 450 pounds. All told, when shipped, the hab weighs 1,550 pounds and can easily fit into a single Twin Otter plane.
Twin Otters (i.e. De Havilland DHC-6 Twin Otter) are ubiquitous in the arctic and Antarctic and are used for ferrying people and/or cargo in and out of short, difficult landing sites. By a curious coincidence the habitat, when stowed for shipping, takes up 384 cubic feet – which is precisely the listed useful cabin volume for this plane. Clear evidence that someone has been paying attention to polar logistics. The weight is also in line with what you can fly with a crew and perhaps several passengers. The main habitat is actually two modular units that are connected together. Additional modules can be added if a longer habitat is desired. The air lock is added at one end and a large “garage door” is located on the opposite end.
The structure is formed of a series of joint inflatable ribs that are surrounded by an external inflation package. The structure is quite strong and is only pressured to about 1 psia over ambient air pressure, Inside the structure are a series of connectors to which internal storage shelving can be added. The floor is presently covered with the commercially available recycled foam rubber matting many people use in their garages at home.
As you look at the structures outside you will see a series of white fan-shaped patches. These are “load patches” and they serve as the place where the guy wires attach to the hab. Upon close examination you can see that these load patches contain four ribs of stronger material sewn in between the load patch and the underlying fabric. This allows the stress exerted on the structure by the guy wires to be more evenly distributed.
The hab is held down by a series of guy wires that are connected to ground anchors. In Delaware, the demonstration used large metal stakes pounded into the ground. In the Antarctic, holes will be drilled into the ground down into the permafrost, anchor units will be inserted, and the holes filled with water that will then freeze, holding the anchors firmly in place. A compacted gravel bed will be prepared for the location where the hab will be placed. The module will be placed directly on this gravel bed and not on a raised platform, as is usually the case with semi-permanent polar structures.
Along the lower portion of the left and right sides of the hab are a series of bags – “regolith holders”. The idea here is to see how rubble could be used as a radiation shield on the Moon. Ideally the entire structure would be covered with a layer of regolith. These regolith holders will be tested out with snow during this initial trial to see how they affect the overall performance of the structure.
Data, Sensors, and Webcams
Since this is a technology demonstrator, ILC wants to be certain that it knows what is happening at any moment to the hab structure. As you walk around inside you can see multiple square patches and other things embedded inside the structure itself. Among the embedded sensors are accelerometers and strain gauges that will allow ILC to monitor the loads the structure encounters as it endures the extreme, and often fierce Antarctic weather. There are also temperature, pressure, CO2, smoke sensors as well. In addition, there will also be a webcam inside the hab and one outside the hab. Both can be remotely swiveled. There is also a weather station located outside the hab.
All of the data collected by the various sensors and equipment in and around the hab goes to a laptop computer. That computer runs the hab systems and sends data back to NASA and ILC during off peak data use times at McMurdo Station. Although the Internet communications at McMurdo there are adequate, they are in heavy demand. Data connections as well as power are supplied directly to the hab by the utility system at McMurdo Station.
Ideally, the hab should operate on its own for the entire year – however it is likely that things may have to be checked from time to time. In those instances, personnel already on site at McMurdo Station will attend to these needs. The hab will be located immediately adjacent to a number of existing structures at McMurdo Station.
This is similar to how the Arthur Clarke Mars Greenhouse has been tested on Devon Island since 2002. There is a period of time in July when humans are in base camp and the greenhouse is checked over, upgraded, etc. However, for the remaining 11 months of the year, the greenhouse operates on its own with the only human interaction being via satellite link.
Planetary Analogs
This is more than just about technology. It is also about operations – and learning. One way to do your homework on Earth – before you leap to other worlds – is to find places that serve as analogs for operational situations and/or environments we expect to find on other worlds.
These planetary analogs are not perfect replicas for extraterrestrial locations. Only those extraterrestrial locations can offer that fidelity. But these analogs do allow scientists, engineers, and managers a chance to chip away at large chunks of the challenges that still confront us as we design the systems that will allow us to work and thrive on other worlds – and do so here on Earth. Antarctica and the arctic offer many planetary analogs for use in testing out new hardware and operations. Some people might ask why NASA would go to the expense of shipping this hab module to Antarctic when you could test it more easily in a parking lot at a NASA center. True. And if something breaks, you can fix it easily. Also true.
Herein lies the reason why going to Antarctica or some other remote location is of such great value – and I have seen this personally from my times on Devon Island. Being there is more important that thinking about being there. If I am going to design something that is going to be robust, resilient, redundant, and self- reliant (ideal attributes for something that is going to the Moon or Mars), I can get to a point intellectually where I probably have a lot of that embedded in a design. But no matter how much intellectual effort I put in to it I know – instinctively and subconsciously – that if something breaks I can fix it.
Now suppose I invest as much brain power as I can into the design and fabrication of my project – but instead of knowing that I can drive to a hardware store – I now know that I have to have every resource I might need with me – and that my resources will be limited so I need a design that will not need to be fixed – or can be fixed easily. Again, that’s fine until you actually take your design to the nastiest, most remote and unforgiving place you can think of and give it a chance to prove itself.
Things will happen that you did not anticipate. A hardware store solution is not what you need. You need to think of something on the spot. This need to innovate is never as pure or strong when you have a crutch to lean back on back home. If you are in a situation where personal risk as well as engineering risk is a real factor, then you find that clarity of thinking to be even more apparent.
Using the reality of being in a real, operationally risky location is what makes all the difference since it serves as an experiential crucible where reality beats your design into a better form. Fixing broken fenders on lunar rovers, capturing broken satellites on the shuttle, repairing Skylab – and of course Apollo 13 – all of these things required on the spot thinking to solve interlaced engineering and operational problems.
To be certain it is just plain dumb to deliberately put you and your expensive hardware into a situation where you are either ill prepared or know things can be dangerous. But if you are designing something to operate for long periods of time in very challenging environments, you cannot get a full appreciation as to how it will perform – and how you will keep it performing – until you push the envelope, so to speak.
Lessons Learned
On Devon Island, we did not set out to build a structure using materials that you’d use in a greenhouse on Mars. That was not what we were interested in learning. Instead, we needed a robust greenhouse – on Earth – one that would survive in a nasty environment that we could operate as if it were on another planet. As such, researchers outfitted it with sensors and computers so as to allow it to be operated remotely – including the growing of plants. But if the researchers decided not to communicate with it – or communications broke down – the greenhouse could take care of itself. That is what you’d want to have sitting on Mars before a human crew arrived or perhaps between human visits. What you make the structure out of for use on Mars is for someone else to experiment with.
In this case, the planetary analog we sought was an isolated remote place that was part of a research station so that we could get infrastructure support. Other people go to Devon Island for another planetary analog that this location offers – a large impact crater – with the operational aspects being of secondary importance to them. And then there are some people see the value of doing real research – in a crater – in a remote location as being of value – not just for the sake of the intrinsic science – but also for operational lessons as well. These opportunities are offered in abundance in Antarctica as well – singly – or overlapping.
As such, planetary analogs can overlap as well as they can stand-alone. The important factor is to know what questions you need to have answered – and which aspects of a planetary analog help you answer those questions and which ones will not.
Taking this hab module to McMurdo Station should provide NASA, ILC, and the NSF with a lot of valuable data. Hopefully they will be able to invest the lessons learned in even more advanced hab units – and then push the envelope even further by placing it in a stand alone situation where it not only has to function on its own – but does so for a full year with of physical human presence. That could be followed by humans actually spending a year living in such a facility – again, all on their own – just like NASA wants to do on the Moon.
Meanwhile in a vacuum chamber somewhere, other teams can start work on how to build a flexible, inflatable structure on the Moon – armed with initial results from these field trials as to how this structure needs to function.
The next step, armed with how to use – as well as how to build – an inflatable lunar habitat might be to have people live inside one for a while as the habitat sits inside the vacuum chamber. Eventually, you’d need to bite the bullet and send one to the moon, set it up, and see what happens – armed with the knowledge that you have done as much of your home work on Earth as you can.
And once you have accumulated enough operational experience on the Moon you can graft that onto how an inflatable habitat might be built for use on Mars.
Sailing ships only improved over the centuries by virtue of being used in actual oceanic environments – first in coastal waters and then on longer voyages. The hardware and systems we use to explore other worlds needs to follow a similar developmental path.