To ISRU or Not to ISRU, This is the Dumbest Question
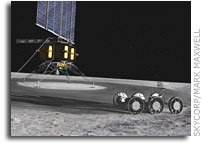
In Situ Resource Utilization or ISRU is the use of materials derived at the location in space that you have traveled to. This is mostly associated with the Moon, Mars, or the Asteroids. Without ISRU there simply is no long term human space program. Why? We simply cannot afford to take everything with us at prices exceeding two hundred thousand dollars per kilogram for the Moon and even more for Mars. Therefore, for any rational human space exploration program, the question is not why but how, when, and where we implement ISRU.
In previous missives (“Bootstrapping the Moon“ and “Plan B For Outer Space“) it has been stated that the purpose of human space exploration should not be primarily science but rather, development. Also, in lieu of NASA’s continuing difficulties in moving outward from low earth orbit (LEO), it was postulated that this would have to have a return on investment, even if over a longer period than a normal venture capital investment horizon. Bootstrapping with as little investment as is practical is the prudent course.
Interestingly enough, the problems of setting up a self-sustaining lunar enterprise mirror in microcosm the problems that we have here on Earth in devising a prosperous, long-term, and sustainable planetary civilization. Therefore, the issues involved can provide lessons as well as possible solutions to our planetary-level problems at home. This becomes a very strong rational for this approach for lunar exploration and development as we now can directly trace from the Moon to practical solutions on Earth. This answers one of the prime political complaints about space exploration: that it does not help to solve our problems “back here on Earth”.
Energy and Lunar Base Site Selection
The key to everything, whether on Earth or the moon, is energy. Since there is no air or fossil fuels on the Moon, what we consider as “alternative energy” sources on Earth such (solar or nuclear) are primary sources on the Moon. For cost consideration purposes, and for the paperwork hassle, nuclear power on the lunar surface is out, at least in the early days. This can be debated an infinitum but for our purposes here, solar is the choice. However, no matter what, energy on the Moon, at least in the early days, is not going to be cheap.
NASA Administrator Griffin has stated multiple times that he wants to buy services from commercial industry for use by the lunar outpost the agency wants to build. We could take him at his word and provide power with NASA buying it from a consortium, thus providing a means of early Return on Investment (ROI). NASA itself, for its own needs, is not going to need as much power as any ISRU-focused system would require. However, some early ROI would be a very good point for any interested party or consortium of private interests as far as spurring lunar development is concerned.
There is a conflict, however, between the best site for a lunar base as far as NASA’s science interests are concerned and the best site for overall development. NASA’s current baseline location for a base is a site on the rim of Shackleton crater near the lunar south pole. This is due to intrinsic scientific interest in the site due to its location at the edge of Atkin Basin, the largest impact on the Moon. It is at this location which scientists believe excavated rock formations that can give information on the formation and evolution of the Moon.
Another reason for choosing this location is that it is very good for ISRU purposes given that the floor of Shackleton crater may have extensive deposits of water ice and or hydrocarbons (so there are some “fossil” fuels there possibly). Some lunar scientists think that between the north and south pole of the moon that there is up to 10 billion tons of ice. If true, that is enough to completely change the equation for economic development of the moon. However, Shackleton crater has significant disadvantages over the lunar north pole.
Recent orbital and radar studies have shown that the terrain at the lunar south pole is incredibly rough. This is understandable inasmuch as the entire region is in an immense crater (Atkin Basin). Also, from a development perspective, the distance to the lunar Mare (the smoother plains regions of the Moon) is several hundred kilometers farther away than they are from north polar regions. Mare Frigoris is 24 degrees from the lunar north pole whereas Mare Nubium is 60 degrees from the lunar south pole. This can be seen in figure 1:
Figure 1: Lunar Polar Projection Maps
The circumference of the Moon is 10,864 km (6,790 miles). This means that the minimum distance between the south pole and the mare is 1,810 km (1,090 miles) while the distance from the north pole to the mare is 724 km (436 miles). Why is this important? In a phrase, lunar development, lunar mare resources, and a lunar railroad. It is incredibly expensive in terms of fuel to take off and land (or to hop) long distances on the lunar surface. Eventually, as lunar development proceeds, a railroad will be a crucial factor. The much shorter distance and easier terrain using a northern polar location is obvious. As an exercise, look for a route on lunar topo maps and see for yourself which is easier.
Another crucial aspect as to whether the Moon’s north or the south is chosen for the first outpost is illumination. Currently, we know that in the northern summer that there is 100% solar illumination on the northwest rim of Peary crater. We know now, based upon recent SMART-1 data, that there is 100% illumination in the south during the summer along the rim of Shackleton crater. During the lunar “winter” we know that in the south there is only about 70% illumination at Shackleton and a few other locations. This is shown here in figure 2:
Figure 2: Illumination at Lunar Summer (north) and Lunar Winter (south) Images courtesy Dr. Paul Spudis and Ben Bussey (www.spudislunarresources.com)
In reality, until we have a year or so of high resolution global imagery from the Lunar Reconnaissance Orbiter, we are not going to have more than a rough idea as to whether the north or the south polar region is the best place to put the outpost. It is absolutely the case that with the mare regions, the shorter distance from the north pole offers a greater opportunity for development at least with valuable known mare resources. Examples include: Thorium (reactors), Titanium (structures, oxygen, and spaceships), and the ability to travel long distances with less difficulty than was the case with Apollo lunar rovers. A location in the northern regions of the lunar surface with year around illumination it will be very valuable real estate for setting up a low cost power system, which is the key to robust lunar development.
Implementing Lunar Solar Power
With solar power on the Moon as the baseline, and with at least the chance of 100% winter and summer solar illumination, what kind of power system would you want – and what would it look like? First of all, any power system that is going to be used to extract and produce oxygen and metals is going to take a lot of power. There is a certain lower threshold of power that you need to have or it’s just not worth doing. After studying many ISRU methods and lunar base ideas, that minimum is at least one megawatt. Anything less just really doesn’t get you enough power to make a lot of things, and making things is why we are there.
One megawatt allows you to use any of the ISRU processes that have been proposed to make oxygen and gives you the power to feed the processes that make metal as well. Most of the processes actually require more power but with a megawatt the minimum is assured and a bootstrapping process can proceed from there. With a megawatt of power several hundred tons per year at a minimum of oxygen can be made, and if the right process is chosen, hundreds of tons of metals.
Figure 3 shows our idea for what we term a “Powerlander”, a self-contained lander with solar arrays and a power processing system to deliver 480 volt, three phase power for lunar industry and human use:
Figure 3: Powerlander on Descent, Rim of Peary or Shackleton Crater (picture courtesy Skycorp Incorporated)
If we use the maximum payload of a Delta IV Heavy (~3600kg) to the lunar surface and use the most advanced (33%) solar cells available, we can get solar arrays that can produce 250 kilowatts – plus the inverter. This would use the series II standard lander described previously. These numbers work out with hardware already available for solar power projects on the Earth that would then be modified for the Moon. Also, choosing a standard voltage like 480 volt, three phase allows for smaller conductors.
To get a megawatt of power you need four of these 250kw-supplying landers. This actually helps to lower the cost a bit inasmuch as they are identically configured. In order to guarantee that a megawatt gets landed you need to actually build and fly five of them, for a total of 1.25 megawatts. This gives a safety margin and also allows the system to fully power an outpost as well. Margin is a good thing for a lunar outpost. With a megawatt of power the lunar installation is now in a power-rich condition that is a firm prerequisite for any serious lunar development.
Cost
There is just no way around it, the megawatt of solar power (1.25 if all landers are successful) is not going to be cheap. Aerospace-grade solar cells are necessary in order to reduce the weight of the system to the point to where 250 kilowatts can fit on a series II lander. This lander is the largest that a Delta IV Heavy (or Ariane V or Proton) can send to the Moon.
A few numbers were run with lightweight silicon solar cells and 19% cells . You can’t use the 21% cells due to the lack of charge dissipation capability. You increase the number of launches from 5 to 9 and with a nominal $200 million per launch you really don’t save any money. Also, the solar cell degradation is a lot higher than with the aerospace grade cells. Table 1 gives a ballpark costing of this system.
Item | Cost | Total Cost |
Launch Vehicle | $200 million each for five | $1 billion dollars |
Lander | $100 million each for five | $500 Million dollars |
Solar Arrays + Inverters | $140 million each for five | $700 Million |
Total | $440 million each for five | $2.2 billion dollars |
Table 1: ROM Cost Estimate for Megawatt Class Lunar Power System
While these costs are non-trivial they do indicate what could be done for a lunar power system if it was done commercially with the excess power sold to the government outpost. It would be nice if this was all there was to it – but unfortunately this is not the case.
A huge issue is the blasting of the lunar surface by these landers. Ideally, you would want to land these landers close to each other. But without some extra work this is not practical. During the Apollo era the second human landing mission (Apollo 12) landed about 255 meters (508 feet) from the Surveyor III spacecraft. One of the goals of that mission was to perform a precision landing, which was accomplished. Another goal was to obtain a piece from the Surveyor III, return it to the Earth, and examine it to determine what the long term effect would be for hardware left on the Moon. Figure 4 shows the Surveyor III spacecraft with the Lunar Module in the background, along with a topographic map of the area:
Figure 4: Surveyor III, Apollo 12, and the Topographic Map of the Landing Site
When the Surveyor III camera was returned to Earth and examined it was found to have been blasted by the landing of the Lunar Module with some particles hitting at over 40 meters per second. This is where NASA is having a problem these days as it ponders lunar surface logistics. At this time NASA is looking at landing the LSAM landers up to a kilometer away and doing things like putting wheels on the landers or other things. This just makes the logistics of the base much more difficult than they need to be.
So we have an interesting problem. Either you land the Powerlanders far from each other and put wheels on them to drive them to each other (a waste of lander payload mass) or you find another solution.
The solution comes from Dr. Larry Taylor of the University of Tennessee, who found that when real lunar regolith (as opposed to the simulated stuff) is placed in a microwave oven, that the material heats to melting temperature faster than water heats to boiling on Earth! With the accomplishment of exposing regolith to temperatures over 1,200 degrees C a whole new ideaspace for working with lunar regolith is opened up.
Drs. Taylor and Tom Meek actually designed a very spiffy rover or trailer for a rover that could be used to actually pave the lunar surface. This is shown in figure 5, along with a microscope sample of the melted surface that results.
Figure 5: Lunar Paver and Sintered Lunar Regolith
A note to be made here is that if we did not have the lunar materials brought back by the Apollo missions we would have not known about this attractive feature of the lunar regolith. Dr. Taylor and his collaborators developed the theory, proven in their laboratory study of Apollo 17 soil, that all mature lunar regolith (which will be abundant at either lunar pole) has a patina of reduced iron, produced from the vaporization of regolith by micrometeoroid impacts. It is this patina that couples extremely well to microwaves such as those put out by a standard microwave oven.
Figure 6: Lunar Paver Concept Vehicle (image credit: Skycorp/Mark Maxwell)
What this means is that one other mission has to be flown after the first Powerlander lands and before the other Powerlanders land on the surface. This would be an automated rover that has a blade and microwave power system on it, along with as much electrical wire as possible, be landed on the Moon. It is then connected to the first Powerlander (it could drive over from its lander a couple of kilometers away) and then be used to sinter further landing areas for the following Powerlanders. The sintering process can be used to make landing pads, berms, and even roads in preparation for human missions. A possible configuration of landing pads and berms is shown in figure 6.
Figure 7: Lunar Paver Concept Vehicle Sintered Landing Pads WIth Power from Lander (image credit: Skycorp/Mark Maxwell)
A rough estimate of the cost of this mission is about $250 million dollars using a series II lander (about 2,000 kg to the lunar surface on an Atlas 551). The rover/paver would only weigh about 500 kg with the rest being the electrical wiring needed for the paver. The paver would have an input power to the microwave system of between 50-100 kW, depending on how fast you would want to do the sintering.
An option that may drive this to the Delta IV Heavy (3,600 kg payload and $320M dollars total) would be if you put an option for a trailer that would collect the volatiles from the sintering process. A 1,200-1,400 C sintering process would liberate all of the volatiles. With more than five acres total sintered in this fashion, the amount of gasses would be several tens of kilograms of oxygen, hydrogen, nitrogen, and other trace gasses.
Figure 8: Lunar Installation with Sintered Landing Pads and Berms (image credit: Skycorp/Mark Maxwell)
Prelude to the Big Time
This is just starting to get to the good part of ISRU. In order to have an efficient ISRU system there is a lot of preparatory work that has to happen. Up until now, we had to start with the philosophy, and then develop the rules of the systems engineering process. Now we are just starting to get to the core value of ISRU: the processing of hundreds and thousands of tons of material so as to obtain volatiles, bulk oxygen, and then metals.
There is purpose to proceeding in the way that these serialized missives have been published: to help to build a gestalt of the systems architecture and engineering that are based upon a completely different underpinning than a mission architecture that is based upon science or as some have called the ESAS lunar phase “touch and go” on the way to Mars. The Moon is intrinsically valuable to humankind in its own right as it will be the first place that we learn to live off the land away from our ancestral home. Mars beckons in the future. But without the Moon, Mars at this time in our history is a bridge too far, just like Apollo was 40 years ago.