Early Mars: Oceans Away?
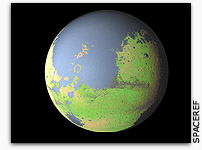
During the 1970s, photos from the first Mars orbiters showed dry river channels that were apparently quite ancient – dating from the first 500 million years of the planet’s existence. The river valleys implied liquid water, raising the possibility that life might have developed.
Today, the Martian surface is cold and dry, and astronomers and climate modelers have struggled to explain such warm conditions in the early years, when the sun was considerably weaker. The leading theory is that early Mars had a thicker atmosphere, which generated a potent greenhouse effect. But to some researchers, this idea seems insufficient to account for above-freezing temperatures.
Owen Toon, of the laboratory for atmospheric and space physics at the University of Colorado, says greenhouse gases cannot explain the presence of liquid water on early Mars. “There was no way for Mars to ever have had this warm, moist greenhouse climate,” he says.
An alternative explanation for the early erosion that made river valleys is colossal energy releases from asteroid impacts. About 25 craters more than 100 kilometers in diameter are visible on Mars, says Toon, reflecting a period of titanic asteroid collisions in the early solar system.
A new model of these impacts on Mars, described by Toon, Teresa Segura, and coauthors in Science (Dec. 6, 2002), paints a picture of a planet where conditions see-sawed between frigid and torrid. Instead of the slow and steady warmth of an enhanced greenhouse effect, this model shows a planet much less hospitable to life.
Toon says that, between impacts, early Mars was “a big frozen iceball.” But after the impact of an asteroid 50 to 250 kilometers (30 to 155 miles) across, the atmosphere would have filled with vaporized rock hotter than 1,000 degrees C (1832 degrees F).
“Within weeks or months, depending on the asteroid’s size, the rock vapor condenses and forms a layer of rock on the surface,” Toon says. Because both the asteroid and the impact zone probably contained ice, the atmosphere also fills with hot steam, causing several years of rainfall.
According to the calculations of Toon’s group, this water caused erosion and left evidence of rivers, lakes and small oceans on Mars. But the hot, wet conditions were ephemeral: the planet froze within a few decades or centuries. Only after the collision of another asteroid, perhaps 10 to 20 million years later, did the warmth return.
Although the detailed calculations of Martian conditions are new, the possibility of a link between impacts and erosion features is not. Via email, Vic Baker, head of the Department of Hydrology and Water Resources at the University of Arizona, said several researchers during the 1980s noted that large, early asteroid impacts “would induce hydrothermal phenomena and could produce small valley networks.”
But the asteroids don’t deserve all the credit, Baker added, especially for the younger valleys. “That is why Gullick and I, and others, have favored volcanic-induced climatic changes for some valley formation.”
All in the Timing
Mike Carr, a Mars specialist at the U.S. Geological Survey, says the impact theory suffers from a “timing problem.” The ancient craters that Toon and company used as putative sources of energy look much older than many of the valleys they supposedly created. “There are simply no large impacts that are as fresh and well preserved as the valleys,” he says. In other words, if the accumulation of erosion is an indicator of age, the craters, many of them almost entirely filled in, must be much older than the valleys.
Carr would not entirely discount impacts as a source of heat, however. “It does not invalidate the basic premise of impact craters as possibly causing the conditions, it just has to be a rain of much smaller bodies, not the very rare, large impacts” that Toon and the others studied. “They are simply not contemporaneous with the valleys.”
Another argument against the impact theory concerns erosion on the rims of some ancient craters, which seems to indicate that the craters are older than whatever caused the erosion. Not necessarily so, Toon says. “Even if that impact happened to form the crater in question, the impact comes first, and the crater comes second. You get 10 to 100 years of flowing water after the formation, so the erosion could have occurred immediately after impact.”
In terms of astrobiology, the impacts produce “a very different scenario for the origin of life,” says Toon. “You just don’t have long, warm period of abundant liquid water. You still have liquid water underground, so you could still have life deep in the interior, but things are not pleasant on the surface.”
If the impact scenario is correct, the river valley networks are not a good place to look for evidence of life, Toon says. “It’s just not the right place; they are probably ephemeral.” A better place, he says, are the much younger gullies, which were apparently fed by hydrothermal water. “They tapped into underground aquifers for a long life, maybe millions of years,” Toon says.
The best way to solve the dispute, Toon says, would be to land on the planet. “The most fruitful way to prove impacts would be to examine thick layers of material that the valleys cut through.” If they are remains of standing water produced under the greenhouse scenario, he says, “they should show a normal rate of dust infall. It would take millions of years, the sediment might look heavily processed by water, and the particle sizes would be small.”
If the layers were composed of hot rock that fell after asteroid impacts, however, they would have “been laid down pretty quickly and melted out groundwater, so you might expect an association with some sort of groundwater release.” The particles themselves would also be different, he adds. “You would see shocked quartz and pieces of meteorite.”
The distinction, he says, could be made pretty quickly, upon close examination, but it may take a space-suited geologist with a rock hammer. “I don’t think an orbiter will tell us. You have to sample the layers.”