Precursor Measurements of Mars Needed to Reduce the Risk of the First Human Mission to Mars
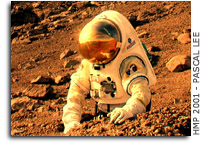
An Analysis of the Precursor Measurements of Mars Needed to Reduce the Risk of the First
Human Mission to Mars
Full Document 1.2 MB PDF
David W. Beaty (Mars Program Office-JPL/Caltech), Kelly Snook (JSC/NASA HQ), Carlton Allen (JSC), Dean Eppler (SAIC), Bill Farrell (GSFC), Jennifer Heldmann (ARC), Phil Metzger (KSC), Lewis Peach (USRA), Sandy Wagner (JSC), and Cary Zeitlin (Lawrence Berkeley), on behalf of the Mars Human Precursor Science Steering Group (MHP SSG)
June 2, 2005
This report has been approved for public release by JPL Document Review Services (CL#05- 0381), and may be freely circulated.
Executive Summary
The Mars Human Precursor Science Steering Group was chartered by MEPAG in June 2004 to analyze the priorities for precursor investigations, measurements, and technology/infrastructure demonstrations that would have a significant effect on the cost and risk of the first human mission to Mars. Based on this analysis, the MHP SSG proposes the following revised phrasing for MEPAG’s Goal IV, Objective A, and within it the investigations that follow (in priority order). The measurements needed to carry out these investigations are described in the subsequent sections of this white paper.
All of the measurements listed below, which are listed in priority order by the degree of impact on risk reduction, would have value to planning the human exploration of Mars (and most particularly the first human mission to the martian surface, for which our lack of knowledge will be greatest). However, the authors of this report are not in a position to determine how much risk needs to be removed in order for the first human mission to be judged acceptably safe. Thus, we cannot a priori determine how many of these measurements need to be successfully completed (i.e. required) before the first human mission can fly. Obviously, a larger precursor program will reduce the risk more than a smaller precursor program. However, the decision on safety thresholds must be deferred to others.
Recommended revision to MEPAG Objective IVA.
Objective A. Obtain knowledge of Mars sufficient to design and implement a human mission with acceptable cost, risk and performance.
The following four investigations are of indistinguishable high priority.
1A. Characterize the particulates that could be transported to mission surfaces through the air (including both natural aeolian dust and particulates that could be raised from the martian regolith by ground operations), and that could affect hardware’s engineering properties. Analytic fidelity sufficient to establish credible engineering simulation labs and/or performance prediction/design codes on Earth is required.
1B. Determine the variations of atmospheric dynamical parameters from ground to >90 km that affect EDL and TAO including both ambient conditions and dust storms.
1C. Determine if each martian site to be visited by humans is free, to within acceptable risk standards, of replicating biohazards which may have adverse effects on humans and other terrestrial species. Sampling into the subsurface for this investigation must extend to the maximum depth to which the human mission may come into contact with uncontained martian material.
1D. Characterize potential sources of water to support ISRU for eventual human missions. At this time it is not known where human exploration of Mars may occur. However, if ISRU is determined to be required for reasons of mission affordability and/or safety, then, therefore the following measurements for water with respect to ISRU usage on a future human mission may become necessary (these options cannot be prioritized without applying constraints from mission system engineering, ISRU process engineering, and geological potential):
The following investigations are listed in descending priority order.
2. Determine the possible toxic effects of martian dust on humans.
3. Derive the basic measurements of atmospheric electricity that affects TAO and human occupation.
4. Determine the processes by which terrestrial microbial life, or its remains, is dispersed and/or destroyed on Mars (including within ISRU-related water deposits), the rates and scale of these processes, and the potential impact on future scientific investigations.
5. Characterize in detail the ionizing radiation environment at the martian surface, distinguishing contributions from the energetic charged particles that penetrate the atmosphere, secondary neutrons produced in the atmosphere, and secondary charged particles and neutrons produced in the regolith.
6. Determine traction/cohesion in martian soil/regolith (with emphasis on trafficability hazards, such as dust pockets and dunes) throughout planned landing sites; where possible, feed findings into surface asset design requirements.
7. Determine the meteorological properties of dust storms at ground level that affect human occupation and EVA.
1. Introduction and Background
1.1. General
Over the past five years or so, there have been two major studies of the robotic program needed to serve as a precursor to the first human mission to the martian surface: MEPAG [2001; revised 2004] and the NRC’s Safe on Mars report [NRC, 2002]. Although these studies are both important, for several reasons it was necessary to reconsider them. NASA’s new Vision for Space Exploration (announced January 2004) includes a number of important planning details that previously had been missing (e.g. general objective, timing and budget), and requires reconsideration of precursor priorities. In addition, the recent discoveries of the unprecedented orbital campaign at Mars, with the Mars Global Surveyor, Odyssey and ESA Mars Express missions, as well as the extraordinary, and surprising findings of the Mars Exploration Rovers on the surface of Mars, have provided a rich set of data that has dramatically altered our understanding of Mars, in a way that both enhances the feasibility of conducting human missions to Mars (i.e., the apparent abundance of near-global, subsurface water, at some depth), while also presenting new potential hazards that must be mitigated (i.e., particularly the increased possibility of biohazards if liquid water is found to be present, and accessible, below the martian surface). Finally, the NRC study was limited to hazards related to the martian environment, and mission planning also needs to include risks of other kinds, as well as precursor technology demonstrations and infrastructure emplacement.
The MHP SSG prioritizations also differ from previous roadmapping activities (e.g. the Bioastronautics roadmap, where risk priorities were solely based on potential impacts to crew health, possibly long term) for two reasons. First, the MEPAG analysis addressed overall mission risks, including system and hardware risks, not just risks to humans. Second, the MHP SSG ranked risk priority based on evaluation of how Mars flight measurements could buy-down that risk. Even if risk was high, if measurements could not buy it down, then priority ranking decreased.
For all of these reasons, in 2004, MEPAG chartered the Mars Human Precursor Science Steering Group (MHP SSG) to reconsider the description of its Goal IV (Preparation for Human Exploration). The charter for the MHP SSG is attached to this report as Appendix 1. In summary, the purpose is to determine, in priority order, the ways in which the risk of a human mission to the martian surface can be reduced by means of flight missions to Mars. In carrying out its charter, the MHP SSG was organized into two major teams: The Measurements subteam, and the Technology/Infrastructure subteam. This white paper represents the report of the Measurements subteam.
Because human missions to Mars will face a large number of risks, it is easy to think of LOTS of precursor investigations and demonstrations that will have some effect on risk reduction. However, the size of the impact of different precursor efforts on risk reduction is far from equal. In a cost-constrained program, it is important to understand these relative priorities. MEPAG measurement prioritizations presented here can be integrated into broader planning activities for human missions by outlining high priority measurements to be made on upcoming missions (both lunar precursor testbed missions and robotic missions to Mars).
1.2. Assumptions
- Assume that there will be a series of robotic missions to Mars, of as yet unknown character and timing, that will be capable of carrying out investigations and measurements, and doing technology/infrastructure demonstrations.
- Assume the first dedicated robotic precursor mission is scheduled for flight in the 2011 launch opportunity, and that the first human mission is scheduled in approximately 2030.
- Assume that a separate sequence of Mars missions, with a primary objective of robotic scientific exploration, will be carried out in addition to the human precursor sequence.
- Assume that the infrastructure associated with the science missions (e.g. the telecommunications infrastructure) is available for use by the human precursor missions.
- Assume for the purpose of this analysis that the 2030 mission and all of the precursor missions are funded by NASA without financial support from international partners.
- Assume the first human mission includes a landed human element.
- Assume that both the “short-stay” (~30 sols) and “long-stay” (~300 sols) missions are still under consideration; separate analyses of the precursor program are needed for each.
- For the purpose of this study, an analysis of the precursor program needed to support only the first human mission to Mars has been developed. The timing and character of human missions beyond the first one are too uncertain at this time. Obviously, additional precursor investigations may be needed to support recurring human missions.
- The human site will have been certified for landing safety with data from robotic missions before the humans land.
1.3. Scope
The scope of this analysis is the investigations of Mars by precursor robotic missions for the purpose of reducing mission risk/cost and increasing performance of a human mission to Mars. The authors note that in order for the program to be complete, the following kinds of precursor investigations also need to be considered, but they are considered within this analysis only to the extent required to directly support robotic flight missions.
- Investigations that can be carried out at the Moon.
- Investigations that can be carried out in Earth-based laboratories or in Mars-analog field environments on Earth (other than on returned martian samples).
- Investigations of the space environment of relevance to a human mission other than those specifically at Mars.
1.4. Definitions
- Soil. The unconsolidated material at the surface of Mars, without implication as to whether it contains an organic component. As used in this report, this term encompasses the following three components: dust which is widely circulated, presumably homogeneous, and fine grained; dune material which presumably is coarser grained and may not be globally mixed and may not account for a large fraction of material; and the regolith which includes material derived from bedrock with an unknown size frequency distribution and origin (presumably a combination of physical and chemical weathering).
- Acronymns: All acronyms are listed in Appendix 2.
NOTE: This document is intended to support future planning, and as such it mentions possible future flight projects that have not been approved. NASA’s flight missions can be implemented only after they have achieved compliance with National Environmental Policy Act and Council on Environmental Quality (CEQ) laws. Additionally, some proposed missions would also require launch approval by the Office of the President.