Opening Statement By G. Paul Neitzel: Hearing on NASA’s Space Shuttle and International Space Station Programs: Status and Issues
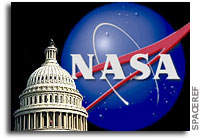
Statement of G. Paul Neitzel, Ph.D. , Professor of Fluid Mechanics, The George W. Woodruff School of Mechanical Engineering, Georgia Institute of Technology
Mr. Chairman, Ranking Member Feeney and members of the subcommittee: thank you for giving me the opportunity to testify on the subject of “NASA’s Space Shuttle and International Space Station Programs: Status and Issues.” My name is Paul Neitzel and I am a professor of fluid mechanics in the George W. Woodruff School of Mechanical Engineering at the Georgia Institute of Technology. I appear today as a member of the NASA external research community. My experience as a principal investigator (PI) on NASA-sponsored research goes back more than twenty years and I have had the occasion to serve the agency in several advisory capacities over that period of time. Of particular relevance to the subject of today’s hearing is my prior service on the Space Station Utilization Advisory Subcommittee (1995-99) and on two groups (in 1999 and 2000) tasked to examine the management of research aboard the International Space Station (ISS). In my written testimony below, I shall address some of the issues I feel are important to ensure that the full promise of the ISS as a research laboratory is realized.
The International Space Station is the culmination of America’s desire for a “permanent” research facility in low-Earth orbit that began with the launch of Skylab in 1973 and continued with the Shuttle-Mir program. The final incarnation of the ISS will be a facility built with the cooperation of sixteen partner nations. The ISS has always been described, among other things, as a laboratory for performing research under the weightless conditions of free-fall, both to enable further human and robotic exploration of space and to answer fundamental questions that could lead to enhanced understanding of terrestrial phenomena, such as the influence and interactions of forces that are often obscured on Earth by the presence of gravity.
NASA’s research program in the life and physical sciences began modestly. The physical sciences program grew out of a program called Materials Processing in Space that sought to explore microgravity to produce materials of improved quality; at the end of the 1980’s only about 70 physical science PIs were receiving funding. In this period, there was pressure on the PI community to develop flight experiments to ensure that payload spaces on the Space Shuttle were full. This pressure led to the development and flight of several (often very expensive) experiments that were either poorly conceived, did not require the weightless environment to answer the research questions, or both, leading to criticism from parts of the scientific community of the quality of NASA- sponsored science.
Beginning about 1989 and continuing through the 1990’s the former Office of Life and Microgravity Science and Applications (OLMSA) made a conscious decision to greatly expand its ground-based research program. The basis for this expansion was to broaden the participating research community to access new ideas and to increase the number of investigations to allow the best, most deserving candidates for flight experiments to percolate up through the pool. This decision served to attract a much broader cross- section of the life- and physical-science research communities to NASA-related research, leading to the development of flight experiments truly worthy of the unique resources provided by the Shuttle and the ISS. At its zenith the budget of the then Office of Biological and Physical Research (OBPR is what OLMSA morphed into) had grown to approximately $1B and the FY03 OBPR Task Book (http://peer1.nasaprs.com/search2003/metrics2003.cfm) shows a broad research program containing roughly 1,000 tasks, supporting over 1,700 PIs and co-investigators and nearly 3,000 students, ranging from undergraduates to postdoctoral fellows. A summary from this document of the numbers of tasks, investigators and students supported is included as Table 1. In my own discipline of fluid physics, which is concerned with the behaviors of liquids and gases, I can state without hesitation that the program supported the very best researchers in the United States and had far surpassed other federal agencies as the principal supporter of fundamental and applied research in the field.
On Saturday, February 1, 2003, the nation watched, horrified, as the Space Shuttle Columbia broke apart upon reentry following a successful research utilization flight to the ISS. Needless to say, the tragic loss of the crew and their vehicle, along with the time lag prior to return-to-flight caused serious slippages in both the ISS assembly sequence and the conduct of research aboard both the Shuttle and the ISS. The progress of research was further exacerbated by the decision to limit the crew size aboard the ISS to two persons, down from three and far short of the full complement of six, further negatively impacting research during the assembly phase.
In January, 2004, President Bush put forth his Vision for Space Exploration (VSE, or “the Vision,” for short), calling for the completion of the ISS by 2010, retirement of the Shuttle at that time and the development of a new Crew Exploration Vehicle (CEV), to be used both for sorties to the ISS and exploration of the lunar surface, returning humans to the Moon between 2015 and 2020. Lunar exploration was further mentioned as permitting astronauts to develop new technologies as necessary a stepping stone to more challenging environments, including Mars. The funding for the Vision amounted to $12B over five years, $11B of which was to come from reallocation of funds within NASA’s existing budget, with a $1B plus-up.
Although the life- and physical-science research program had begun to suffer from decreased funding in the aftermath of the Columbia accident, the transition to the implementation of VSE was its death knell. In December, 2005, NASA sent letters to hundreds of investigators in the program, informing them of significant cuts in their funding for FY06 and the termination of their grants effective September 30, 2006. In line with the Vision, NASA decided that future agency-supported research would be restricted to exploration needs, namely the study of “exploration requirements in human health and countermeasures as well as applied physical sciences for fire prevention, detection, and suppression; multiphase flow for fluids such as propellant; life support; and thermal control applications,” as stated in The National Aeronautics and Space Administration (NASA) Research and Utilization Plan for the International Space Station (ISS) published last year. The small number of projects being investigated, although relevant to exploration, is inconsistent with the conduct of a robust, safe exploration program that will send astronauts to the Moon no sooner than 2015.
During a period with limited flight opportunities and a major shift in mission focus, precisely the opposite should be occurring within NASA’s research programs. I shall focus on the physical sciences for the moment. Long-duration exploration such as a 500 day mission to Mars requires new solutions to long-standing problems. A principal limitation associated with such a mission is the up-mass that can be accommodated with a given launch vehicle. For a 16-day Shuttle mission, a stay aboard the ISS with regular resupply, or even a lunar outpost permitting periodic re-supply, existing solutions to environmental and vehicle needs may suffice. For a Mars mission, however, every kilogram devoted to an environmental- or fire-control system, for example, is one kilogram less that may be used for food and water. New systems for Mars missions will also need to be “dual-use,” in that they will need to be able to function for an extended period of microgravity during flight as well as in the 3/8 g Martian gravity. Hence, new, more mass-efficient solutions need to be sought for such problems and a robust program of both fundamental and applied ground-based research should be able to identify good candidates for subsequent flight testing and development as the ISS facilities become available. The current exploration research plan appears to be focused on the investigation of very limited possibilities for such solutions, and is likely to yield less- than-optimal ones. The decision to rush to development at this stage is hard to understand; in this era of incredibly fast-paced technological development, NASA owes it to itself to keep as many options open until it is absolutely necessary to start “cutting metal.”
Under the 2005 NASA Authorization Act, NASA has been directed to devote 15% of its ISS research funds to non-exploration research. The figure for FY07 includes $3.1M for ISS physical science, $1.7M for ISS life science, $3.9M for research aboard free-flyer vehicles, and $3.3M for ground-based grants, for a total of $12M. This number is particularly small, in part, due to the fact that the 15% is mandated to come from the “ISS research” funds, which are at a depressed level due to the inability to conduct meaningful amounts of research on the vehicle during the compressed assembly sequence. As a comparison point, the $3.3M devoted to ground-based research would support, at minimal funding levels, roughly 30 PIs, a figure that can be compared with the numbers from the 2003 OBPR Task Book that were mentioned above and included in Table 1, namely, roughly 1,000 tasks supporting more than 1,700 PIs and co-investigators and nearly 3,000 students. Furthermore, the non-exploration fundamental and applied research that could be conducted aboard the ISS under a robust research program aligns perfectly with the goals of the American Competitiveness Initiative.
The recent decimation of the external research community has other consequences. First, NASA seems to regard research as a spigot that can be turned off and on at will. I would agree that it is easy to turn off the research spigot at any time, but in doing this, NASA is also shutting down the water-treatment plant that supplies the spigot. The reestablishment of an external research community will take years, if it can be accomplished at all. My colleagues in the life and physical sciences have a variety of research interests for which funding is available through other federal agencies as well as from private industry. The very best members of the research community are moving on to other pursuits and are not likely to be able, if even willing, to reengage in microgravity-related in the future.
Second, NASA is fond of speaking of the current crop of researchers who were motivated to pursue careers in space-related research by their fascination with the Mercury, Gemini and Apollo programs that culminated in landing astronauts on the Moon. With the absence of NASA-oriented research programs in our universities, where will the next generation of these researchers come from? Those of us in the higher-education community are sensitive to the fact that it has become harder to attract, and most importantly, to retain American students to study mathematics, engineering and the sciences. With the loss of motivators such as the possibility of a career in a vibrant, active, space-research program, one more incentive for future students disappears. As mentioned already, the former OBPR research program provided opportunities for nearly 3,000 U.S. students to engage in meaningful NASA-related life- and physical-science research. Other nations, notably China, are increasing their emphasis on space research; Asian cultures, in general, embrace the study of science and engineering. As we stand by and watch jobs and technology being transferred overseas, are we as a nation prepared to relinquish our superiority in space research and in the associated discoveries that can drive new businesses and jobs?
Finally, abandonment by NASA of the ISS as a platform for fundamental and applied external research with both exploration and non-exploration applications sends an unfavorable message to the international partners who have contributed their time and money to make the ISS a reality. How can the United States, in good conscience, turn its back on these partners, not to mention the American taxpayers who have borne the bulk of these expenses? An incredible investment of both time and money has been made, both in the ISS vehicle and in discipline-specific research facilities that are to be flown aboard it. These facilities exist, have already been paid for, and are merely awaiting integration aboard the ISS to be used. We need to ensure that the ISS is fully utilized to the full term of its lifetime, currently projected for 2015. This is only five years past assembly-complete, a period we should certainly be willing to sustain.
Last year, a group of us in the life and physical sciences were asked to come up with a “keep-alive” dollar figure for the life- and physical-sciences research program. The figure we estimated that would be required for PI support was roughly $70M/year. (This is exclusive of transportation costs and NASA Center personnel salaries for flight- experiment support, but these would be minimal during the remainder of the assembly sequence.) This funding level would support a research effort roughly half the size of the research program that was in place immediately prior to the recent cutbacks. This $70M/year amounts to less than 25c/year from each American citizen and less than 0.4% of NASA’s roughly $18B annual budget. This small cost of maintaining an active research community is one that must be borne. The cost of losing the possible accomplishments of an entire community that can likely never be reestablished is far greater.
Mr. Chairman, you have posed several questions in your invitation that you would like me to address in my testimony. I shall respond to each, in turn, although some of these responses will reiterate material covered in these opening remarks.
1. What actions does NASA need to undertake to ensure that the International Space Station (ISS) can be productively utilized for exploration-related and non- exploration-related research once it is assembled? When does NASA need to undertake the recommended actions? Which of the actions would you consider to be the most important to the effective utilization of the ISS?
The principal need to ensure productive utilization of the ISS is the existence of a broad- based research community with projects in the pipeline. In addition to candidate flight experiments, this requires a substantial commitment to a ground-based program so that the previous mistakes made by the agency described in the introduction above are not repeated. Because the process for taking a research idea from conception to flight typically requires several years, NASA is already behind in terms of a time schedule that includes a 2010 assembly-complete date for the ISS. However, given that several projects relating to both exploration and fundamental/applied research were recently active, it may be possible to resurrect some of them in an effort to jump-start the reformation of a broader research community. To re-establish such a community, NASA must commit to the long-term research utilization of ISS; those who were left at the altar by NASA this past time will be uneasy about returning in the absence of such a commitment. Many who have moved on to other pursuits will not be able to return to NASA research due to their new commitments.
2. Does NASA have well defined objectives for utilizing the ISS, and are NASA’s facilities, plans, resource allocations, and research criteria and prioritization for utilization aligned with those objectives?
NASA’s utilization plan calls for a research focus for the ISS that is restricted to exploration goals in support of the VSE. The small number of investigations outlined in NASA’s aforementioned ISS Research and Utilization Plan is inconsistent with an effective, safe exploration program. In the fire-safety arena alone, NASA should be exploring fundamental and applied research in a broad range of the field of combustion to guarantee that the very best techniques for fire prevention, detection and suppression are developed. As pointed out in the recent NRC report entitled, Review of NASA Plans for the International Space Station, the risk of a fire incident on a long-term mission such as one to Mars is high. Not only must spacecraft have the best technologies on-board for prevention, detection and suppression of fires, they must be prepared to rid the spacecraft environment of potentially harmful soot and other products of combustion in the event a fire does occur. Studies of relevance to this also have applications to microgravity dust management and its effect on both humans and equipment functionality. Earthbound applications include clean-room technology, handling of nanoparticles, the detection of nanoparticle health hazards and improved fire-detection equipment.
Mass efficiency of other types of exploration-related hardware is a general concern. For example, NASA knows how to move liquids from one location to another within the spacecraft environment, but are the most mass-efficient means for accomplishing these tasks being developed? The VSE is not limited to the exploration of the Moon, but speaks of missions beyond the Moon, beginning with Mars, for which the up-mass limitations are critical. Even if such post-lunar expeditions are far in the future, NASA should be preparing now by exploring the best-available technologies, and these can only be identified through a robust program of both fundamental and applied research. Lunar expeditions are to explore the possibilities of living on the lunar surface, implying the conduct of research to investigate issues such as in situ resource utilization. Just as mass- efficient spacecraft systems for Martian missions increase the amount of water and food that can be carried on-board, so would mass-efficient systems developed for lunar missions increase the amount of research instrumentation able to be transported, per flight, to the lunar surface.
NASA has available a total of 20 International Standard Payload Racks (ISPRs) for research purposes; 10 in the U.S. lab and 5 each in the JEM and Columbus facilities. Its intention is to utilize half of these ISPRs for exploration-related research and development, but it is difficult to envision that the limited number of investigations described will be sufficient to fully utilize these resources. As mentioned in my introductory remarks, the fact that we are several years away from the required selection of some enabling technologies suggests that a vigorous research program be sustained in order to seek optimal solutions.
3. Does NASA have appropriate plans, programs and resource allocations to ensure that there will be a research community in place and adequately prepared to effectively utilize the ISS once the ISS is assembled?
No. NASA has made the decision to get out of the non-exploration research business and to dramatically restrict investigations related to exploration. The external research community that existed just a few years ago is no longer in place and there are no pending NASA Research Announcements, so the prospects for at least the near future look dim. As also mentioned, this research community has moved on to other pursuits and is engaged in and committed to research that cannot be dropped to return to NASA- related work, even if the community were tempted to do so.
NASA is encouraging the use of the ISS by other federal agencies with interests in microgravity experimentation, however, those agencies are expected to cover the full cost of taking their ideas to flight, including the very expensive transportation portion. I understand that there has been some recent interest on the part of the NIH in partnering in this fashion, but find it difficult to believe that, given budget constraints, a significant amount of research from NIH or other federal agencies will materialize in the near future to enable the full utilization of the 10 ISPRs that will be available for non-exploration use aboard the ISS.
4. What microgravity research in the physical and life sciences is needed to enable future human lunar and Mars exploration missions? Are there any advantages to or requirements for conducting such research on the ISS as opposed to a lunar outpost, on lunar sorties, or on free-flying platforms?
As described above, long-duration manned space flight will require the development of mass-efficient, dual-use hardware to accomplish tasks that are now performed in, perhaps, less-than-optimal ways. The ISS is the only platform that provides access to long-term weightlessness. The challenges in designing for the 3/8 g environment of Mars or the 1/6 g environment of the Moon are routine; for zero-g, however, the absence of buoyancy (the force that causes hot fluids to rise on Earth) provides an environment in which other forces, typically “masked” on Earth by buoyancy, are dominant and exploitable. Lunar outposts will be suitable test beds for some, but not all, technologies designed to work on the Martian surface. For example, the Moon has no atmosphere while Mars does. Therefore, heat-rejection needs in non-habitat situations on the lunar surface must rely on the heat-transfer mechanisms of radiation and conduction, while convection that transports heat due to fluid motion can play a role on the Martian surface. Lunar sorties, although of reasonably short duration, will provide some microgravity periods for experimentation, if crew time permits, but not to the extent that can be performed with a dedicated crew aboard the ISS.
In the life sciences, long-duration space flight poses well-known problems for astronauts, bone loss being a major one for which no existing countermeasures have been effective. Another major hazard, particularly for human exploration of Mars, is the exposure to radiation; the recent NRC report rules out radiation shielding as being up-mass prohibitive and cautions that the exposure experienced during a 3-year Mars outpost mission, “has the potential to produce significant long-term effects that may not be limited to cancer induction.” The NRC panel recommends searching for pharmacological interventions to deal with these effects. Studies on the effects of microgravity and space environments on the entire range of scales within the human organism, from whole-body to cellular levels, seem warranted. The psychological problems of coping with the isolation experienced during a 500 day Mars mission are also of concern. Finally, long-duration space flight also means that astronauts will likely be growing some of their own food, meaning further study of plant cultivation in microgravity is likely necessary for exploration purposes.
The use of free-flyers is definitely a way to get some research requiring a quiescent microgravity environment conducted. Such experiments need to have excellent telescience support in order to both control and retrieve data from the experiment. Those experiments that need human intervention, for example, to change samples, are not candidates for free-flyers. In addition, in the event of an unforeseen occurrence requiring repairs, the free-flyer experiment is typically over. Although careful thought and preparation goes into the design of every flight experiment, things often happen that cannot be anticipated.
5. What are your perspectives on the intended and potential use of the ISS as a national laboratory? What is necessary to enable ISS to be an effective laboratory?
As a member of two task groups that studied the management of research utilization aboard the ISS, I am generally supportive of the concept of operating the U.S. research facilities on board the ISS as a national laboratory. A principal benefit is the buy-in that is likely to come from the external research community to a laboratory managed by a consortium of their peers. This has certainly been the case with the Space Telescope Science Institute, although it is recognized that the degree of complexity of research management aboard the ISS is much larger.
What is disconcerting about NASA’s plans for the national laboratory concept, however, is that there is virtually no funding associated with it, either to support in-house or external research or to provide for transportation of experiments to and from the ISS. Operationally, the national laboratory would serve a role not unlike that of existing NASA Centers, from the standpoint of integrating research experiments into the ISS, but very unlike a NASA Center, in that national-laboratory personnel would not have any discretionary funding to pursue research aboard the ISS without competing for funds from other agencies willing to perform research there. The types of individuals the research community would like to have assisting them with the development of their flight experiments are other researchers who are knowledgeable about the logistical and scientific issues associated with their work. Attracting such individuals to a national laboratory with no provision for even small-scale investigations of their own will be a difficult task. In addition to the national-laboratory funding needed for direct PI support and a modest amount of in-house research, there is a substantial amount required for the development, qualification and integration of experiment-specific hardware for the ISS. These functions are currently supported, to a large degree, by the NASA Centers (which, by the way, typically have discretionary funding to support in-house research). A mechanism for seamless collaboration between the Centers and the national lab or for the transfer of responsibilities (and funding to support them) to the national lab must be worked out.
NASA is supporting the development of Commercial Orbital Transportation Services (COTS) that it hopes will provide transport of experimental equipment to the ISS following assembly-complete. To be sure, transportation to and from the ISS is the “rate- limiting” factor controlling its efficient utilization. There are a variety of payload types used for research purposes, the broad classifications for transportation purposes being pressurized and non-pressurized, with the former obviously a requirement for living systems such as plant and animal models. Certain types of research payloads, e.g., living systems, also require timely transport of payloads both to and from the ISS for the proper conduct of the experiments and analysis of results. It is a leap of faith to assume that a sufficient number of such systems will be ready to perform all the necessary functions with the required frequency at assembly-complete. The failure of COTS solutions would leave the CEV as the NASA backup, requiring the additional purchase of transportation services from Russia, and perhaps from other nations that are talking of developing such systems.
In summary, it is my belief that NASA is ill-prepared to fully utilize the research facilities of the ISS upon assembly-complete in 2010. Faced with the loss of launch capability and a new exploration mission to be funded within its existing budget, NASA has chosen to virtually eliminate its dynamic extramural research program in the life and physical sciences. This move is short-sighted and has caused the loss of a research community devoted to NASA-related issues that will be difficult to reconstitute. Without the existence of this community, there is little to support the utilization of the ISS. The successful implementation of the Vision for Space Exploration will require the presence of an active, diverse research program performing investigations at the cutting edge to define the technologies necessary for successful exploration while, in addition, gaining deeper understanding of fundamental scientific issues that can serve to benefit life on Earth. NASA has always been regarded as a research agency. In this rapidly changing technological world, the need for it to remain a research agency is more compelling than ever.
Thank you again, Mr. Chairman, for inviting me to speak with the subcommittee. I am happy to answer any questions about these issues that you may have of me.
Table 1: Statistics for life- and physical-sciences research for NASA OBPR programs for FY03 (Source: OBPR Taskbook http://peer1.nasaprs.com/search2003/metrics2003.cfm)