NASA Dawn Journal January 27, 2009
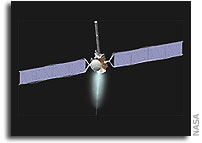
Dawn continues on course for its pas de deux with Mars on February 17. The planet’s gravity will gracefully assist the spacecraft on its way to rendezvous with its intended celestial partners Vesta and Ceres in the more distant asteroid belt. Even the extraordinary capability of its ion propulsion system would not be sufficient for Dawn to complete its celestial dance without the help of Mars.
In the last log we saw that the mission operations team was preparing to adjust the probe’s flight path to keep it on target for next month’s flyby. Just getting to the vicinity of Mars is not sufficient, as the passage by the planet is only one short segment of a very long itinerary. Indeed, choreographing Dawn’s trajectory is a complex matter of finding the most efficient route through the solar system to travel from the moving platform on which it started (Earth) to encounter Mars in just the right way to reach Vesta at the proper time to complete its work there before it has to begin the trek to Ceres to meet it on schedule, aided during most of the journey by the ion propulsion system. Dawn must arrive at Mars on time, traveling in the correct direction and at the necessary location, for the gravitational slingshot to yield the desired effect. Flying the spacecraft through that “window” at Mars is like threading a celestial needle.
Early this month, engineers determined that the craft’s interplanetary route was so close to the one planned originally that it was unnecessary to refine it. In part, this is a reflection of how accurate the first (and now only) trajectory correction maneuver (TCM1 “where do normally undemonstrative engineers come up with these incredibly cool names”) was when it was executed on November 20. In addition however, Dawn does not need to be aimed as accurately as a typical spacecraft might for such a gravity assist. The ion propulsion system will be used so extensively between Mars and Vesta to continue modifying the probe’s orbit around the Sun that it has enough leverage to compensate for a significant range of deviations from the mathematically optimal bending of its course by Mars’ gravity.
To understand the targeting at the red planet, at the request of readers on planets of all colors throughout the constellations Sagitta and Sagittarius, we shall extend the analogy of the archery problem described in an earlier log. We can follow both the spacecraft and the arrow as each travels to its goal. Of course, while the arrow’s path ends when it produces a satisfying thunk at the target, Dawn’s target is a region of space near Mars that is much more penetrable. There are other important differences between the two problems, not the least of which is that Dawn has thrust with its ion drive most of the way to Mars. (For the complete list of caveats that apply to the analogy, contact the Dawn legal department.)
When Dawn was fired into space, aiming for the window near Mars was analogous to shooting an arrow at a target 47 kilometers (29 miles) away. In the center of the target is a red circle 30 centimeters (almost 1 foot) in diameter, representing Mars. Of course, we don’t want our arrow to hit the red bull’s-eye! Rather, our goal is a spot about 2.2 centimeters (7/8 of an inch) outside the circle, near the 11:00 position.
By the time of TCM1 2 months ago, Dawn had traveled 880 million kilometers (550 million miles), corresponding to the arrow having sailed 39 kilometers (24 miles) from our bow. After flying that tremendous distance, our projectile was headed for the bull’s-eye itself, so we applied a tiny adjustment to put it on course for the real aim point.
By January 15, when the mission operations team had scheduled TCM2, Dawn had put more than another 110 million kilometers (70 million miles) behind it, so our arrow would have streaked another 5 kilometers (3 miles) closer to its target. Even with so far still to go, the aim is so good that no further correction is needed.
Although controllers will continue to monitor Dawn’s trajectory and refine the predictions of its course, as long as the arrow flies true, it will reach its target. With the latest estimate, instead of hitting the mathematically optimal spot, it will strike less than 2 millimeters (a little over 1/16 of an inch) farther from the bull’s-eye. It won’t quite land at the 11:00 position, but it will be less than a third of the way to the tick mark just after the 11:00 marker, where the hour hand would be pointing at about 11:04. This is comfortably within Dawn’s allowance for accuracy.
These coordinates correspond to the spacecraft passing about 543 kilometers (337 miles) above the reddish surface of the planet rather than the original plan of 500 kilometers (311 miles). While that may seem like a large difference, the effect of a gravity assist largely depends on the distance from the center of a planet, not the surface. In that context, instead of passing about 3896 kilometers (2421 miles) from the center, Dawn will pass 3939 kilometers (2448 miles) from that reference location. (The arrow strikes 17.4 centimeters, or less than 6 7/8 inches, from the middle of the bull’s-eye rather than 17.2 centimeters, or 6 3/4 inches.) The mission easily could accommodate much larger deviations from the original plan.
And what does the difference mean for the overall journey to the asteroid belt? Dawn’s 4-month period of coasting between Mars and the resumption of ion thrusting will be shortened by about 4 days. As the spacecraft will thrust for most of the 2.5 years from Mars to Vesta, powering up the ion drive a few days earlier is virtually inconsequential.
Over the coming weeks, navigators will continue to gather data on Dawn’s route through space to refine their computations, and some of the numbers above likely will change a little. It might be tempting to think that if we had truly perfect knowledge of the spacecraft’s position and velocity at some time then we could predict the encounter details correspondingly accurately. After all, the gravitational forces from the Sun and Mars and even other planets and asteroids, far though they are from Dawn, are very well known indeed. It turns out, however, that there are other forces acting on the spacecraft as well. They are like a soft but variable breeze blowing on the arrow we have aimed at the distant target, making the already challenging archery problem still harder.
Light, as insubstantial as it feels to our corporeal readers, has momentum, so as the Sun shines on Dawn, it constantly modifies the trajectory. (The force from the light is far greater than from the solar wind, the stream of charged particles that our star blows into space. The effects of the solar wind are too tiny to matter.) The constant nudge from the Sun’s light is small, but it needs to be incorporated into the targeting, and that is difficult because the size of the effect depends on the reflective properties of the spacecraft. Light bouncing off a polished piece of metal provides a different force from light absorbed by a dull black thermal blanket. As the spacecraft changes its orientation, different components are exposed to the light from the Sun, and the angles at which they are illuminated change. Navigators are able to account somewhat for the solar pressure, but the small uncertainty in it contributes to the uncertainty in the encounter details.
Dawn itself is responsible for an even larger variability in its own trajectory. As we have seen before, the spacecraft is equipped with reaction wheels, which are part of the attitude control system. (To achieve a certain mystique about their work, engineers use the term “attitude” to describe the orientation of the probe in the weightlessness of spaceflight; the system also happens to have a very sanguine attitude about its work.) By electrically spinning the wheels faster or slower, the spacecraft can control its attitude (and sometimes even its outlook). The reaction wheels are used to start and stop turns as well as to hold the craft’s attitude steady, right now, for example, keeping the main antenna pointed to Earth. The solar pressure not only alters the spacecraft’s trajectory but it can rotate the spacecraft as well, just as wind can both propel and turn what it blows against. Attitude control automatically changes the wheels’ speeds to counteract this effect. The consequence, however, is that the units gradually speed up as they hold their own against the incessant solar pressure.
The wheels cannot increase their speeds indefinitely, and they lose effectiveness when they reach their limit. To allow them to slow down, attitude control calls upon its colleague the reaction control system to fire small thrusters, which use the rocket propellant hydrazine. The force essentially reverses the influence of the solar pressure that has caused the wheels to spin up, thus allowing them to spin down without the spacecraft ever having to change its attitude. Indulging their usually secretive poetical impulses, engineers refer to this process as “desaturating the reaction wheels.”
In addition to slowing the wheels, the thrust from the hydrazine pushes the spacecraft, modifying its trajectory. During the current phase of the mission, the thrusters are operated twice a week to desaturate the reaction wheels, so engineers need to quantify the ramifications of firing the reaction control thrusters prior to the encounter with Mars. Here again, while the phenomena are understood, there remains some level of uncertainty in how accurately they can be predicted, given imperfect knowledge of the actual effect of the solar pressure and details of how the spacecraft will operate its 6 thrusters to reduce the speeds of the 3 operating wheels.
The uncertainty in the change in the spacecraft’s speed from the desaturation of the reaction wheels is around 1 millimeter/second (12 feet/hour). Except to our readers in the planetary nebula NGC 6543 (sometimes known as the Snail Nebula), this speed may seem very slow, but consider a simplified example of how this affects the calculation of exactly where Dawn will be as it sails past Mars. Suppose the planetary encounter is 5 weeks away, and all present and future trajectory parameters are known perfectly except the result of the desaturation scheduled for 4 weeks before the encounter. (This example disregards all the other desaturations yet to occur, treating them as if they would be known exactly.) The best prediction of the effect on the spacecraft’s trajectory of that desaturation still a week away is included in the analysis to determine where Dawn will be when it reaches Mars, but that prediction is off by just 1 millimeter/second. That speed, when maintained for the more than 2.4 million seconds in the 4 weeks between the desaturation and the flyby of Mars, leads to a distance of more than 2.4 kilometers (1.5 miles). So, if the actual effect of the desaturation is 1 millimeter/second different from what is included in the computations, Dawn’s actual position will wind up 2.4 kilometers away from the calculated position. Of course, all other parameters are not known perfectly, and there are more desaturations before Mars, so the actual encounter conditions can change by much more than in this illustration. Nevertheless, there has been great effort to establish how unpredictable all the factors are, and engineers are confident the trajectory is well within the required window to achieve the needed assistance from Mars.
The powerful tug exerted by the planet will bend Dawn’s path by about 78 degrees. To picture that angle, suppose Mars is a dot at the center of a clock, and Dawn flies toward it (or, more accurately, toward the required window very nearby) from the 12. If the planet had no gravity, the spacecraft would continue in a straight path, exiting the face of the clock by the 6. Instead, the probe takes a sharp turn at the center of the clock and heads out between the 3 and the 4. (This is not the same clock used in the discussion of solar conjunction in the previous log. Be sure to check out the full selection of celestial timepieces in your planet’s Dawn gift shop.)
The deflection from Mars changes Dawn’s orbit around the Sun (as does thrusting with the ion propulsion system). To enter orbit around Vesta, Dawn needs to match its orbit around the Sun to the one that Vesta is in, and the Mars encounter is designed to help accomplish that, bringing Dawn’s plane into closer alignment with Vesta’s. (We saw in the previous log that part of the journey requires changing the plane of Dawn’s solar orbit.) The gravity of Mars will alter Dawn’s orbital plane by about 5.2 degrees, a seemingly modest angle. Yet, if it were up to the spacecraft to accomplish such a change on its own, it would require a velocity change of more than 2.3 kilometers/second (5200 miles/hour).
Thanks to the design of the Mars encounter, there is another benefit as well. In addition to tilting Dawn’s orbit around the Sun, Mars changes its shape, enlarging the elliptical orbit and sending the probe farther from the Sun.
In considering the size of solar system orbits, it often is convenient to measure lengths with the “astronomical unit”, which is simply the average distance between the Sun and Earth. So 1 astronomical unit (AU) is 150 million kilometers (93 million miles), conveniently helping your correspondent locate not only how far he lives from the Sun, but also how far he works from it.
If Mars had no gravity and thus could not divert Dawn on its travels, the craft’s current elliptical orbit would take it as close as about 1.23 AU to the Sun and as far as 1.69 AU away. (For comparison, Mars orbits between about 1.38 AU and 1.67 AU.) As we saw in a log last summer, Dawn is temporarily heading in toward the Sun now. Without the effect of Mars, and with no additional ion thrusting, our interplanetary robot would reach that minimum distance in June 2009, and by early May 2010 it would have swung out to the greatest distance, only to begin falling back again as it followed its elliptical loop.
After the boost from Mars, if Dawn undertook no further thrusting, it would come no closer than 1.37 AU to the Sun, reaching that distance in April 2009. Then it would head out to 1.84 AU, arriving there in April 2010. In this new orbit, Dawn still will be a long way from Vesta (which never dips even quite to 2.1 AU from the Sun) and still farther from Ceres (which travels out to 3.0 AU), yet it will be much closer than it would have been without Mars’ help.
Reshaping Dawn’s orbit, quite a separate effect from reorienting it, would require more than 1.1 kilometers/second (700 miles/hour). The combination of these two benefits is equivalent to the planet imparting about 2.6 kilometers/second (1600 miles/hour) to the spacecraft.
Dawn’s unique propulsion system allows it to change its own speed by well more than this during its mission. Yet the famously gentle ion thrust means it would take quite a while to achieve these changes, and the mission itinerary, fit between the September 2007 launch and the February 2015 arrival at Ceres, does not afford enough uncommitted time. There are other technical reasons as well that making these changes only with its built-in capabilities would be impractical. The encounter with Mars is a free way to get significant help.
Is it really free? Well, in these difficult economic times, there is a cost we are obligated to divulge in the interest of full disclosure. The changes to Dawn’s orbit come at the expense of Mars’ orbit. Just as when you throw a ball forward, you feel a “reaction” force backward, in pushing the spacecraft one way, Mars reacts by moving the other. Mars exerts a force on Dawn, but Dawn exerts an opposite force on Mars. As the planet’s mass is nearly 600 million million million times that of the spacecraft, the effect on our probe is far larger than on the fourth planet from the Sun. The cost of helping Dawn is that Mars will slow in its orbit enough that after 1 year, its position will be off by about the width of an atom. Adding up the growing deficit, it would take 180 million years for Mars to be out of position by 2.5 centimeters (1.0 inches). That is the cost, and, on behalf of Dawn and all who share in the eager anticipation of the mysteries it will reveal in the asteroid belt, we express our gratitude to Mars for its upcoming sacrifice!
The control team’s best estimate now is that Dawn’s closest encounter with Mars will occur at about 4:29 pm PST on February 17. In the next log, we will cover some of the spacecraft’s activities during its short visit, but we conclude this one with a note about the plans. Many spacecraft have visited this intriguing planet already, and it has been studied extensively from orbit and from the surface for years and years. During its brief passage, Dawn cannot learn much that is new. On the other hand, Mars is so well characterized and familiar that it provides a useful reference for calibrating Dawn’s scientific instruments. As we will see, the calibration plan precludes obtaining the highest resolution images that might otherwise be attempted. Such a strategy may seem surprising to all those who appreciate spectacular pictures of Mars, but, of course, there are already many many such images, and future missions will produce even more that will continue to captivate and inspire us.
The instrument calibrations will be a nice bonus, but if Dawn ended up flying by Mars and conducting no calibrations whatsoever, as long as the trajectory targeting were correct, everyone on the project would consider it a success, because the gravity assist is all that is required for the mission to forge ahead to its goal of unlocking exciting secrets deep in the asteroid belt. Dawn’s focus is on preparing for the unknowns of Vesta and Ceres rather than the knowns of Mars.
Dawn is 4.6 million kilometers (2.9 million miles) from Mars. It is 358 million kilometers (222 million miles) from Earth, or 900 times as far as the moon and 2.41 times as far as the Sun. Radio signals, traveling at the universal limit of the speed of light, take 40 minutes to make the round trip.