Independent Analysis of Alternatives To Divert a NEO on a Likely Collision Course With Earth
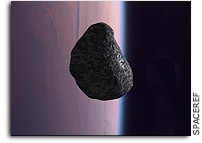
19 March 2007
Mr. Michael Griffin
Administrator
National Aeronautics and Space Administration
300 E St. SW
Washington, DC 20546
Dear Mike:
Based on our recent email exchange and your invitation to, “Send us your specific criticisms, or come in and talk, either way, and we will hear your concerns, and respond” I have put together an independent analysis for your consideration.
What I attach is approximately a parallel analysis of the various alternatives for diverting a threatening NEO to that which NASA submitted to the Congress. In it I provide the basic logic and resultant conclusions and then juxtapose them with the NASA response commenting on why the apparent difference.
Without having access to the NASA Final Report (2006 Near-Earth Object Survey and Deflection Study) there is little more I can do to identify the actual source of the differences. Your team, perhaps in consultation with outside experts, can examine what I present here and decide whether or not the bases for the different conclusions justify any changes in the NASA report.
A few words re what is covered. Neither I personally nor B612 Foundation in general have any specific knowledge of the life cycle cost numbers used in the first portion of the NASA Report to Congress (options for meeting the 140 meter Spaceguard goal) and hence I have little to offer for this section of the Report. The only observations of note are, 1) that the life cycle costs for most of the alternatives considered exceed the equivalent life cycle cost estimates made in the August 2003 NASA SDT report, Study to Determine the Feasibility of Extending the Search for Near-Earth Objects to Smaller Limiting Diameters by a factor of over 2, and 2) that the Venus-like orbiting IR telescope is undervalued in that quite separate from meeting the Congressional 140 meter discovery goal, such an asset dramatically improves the discovery and tracking of the most challenging NEO cohort, those objects in Earth-like orbits and the Aten class of objects in general. Without an interior-orbit telescope there will be large, even decade-long dropouts in the critical tracking information so necessary for deflection decision-making.
The subject of my analysis is the third charge issued by Congress, an “analysis of possible alternatives that NASA could employ to divert an object on a likely collision course with Earth”. In my analysis, with limited time and resources, I have had to make a number of what I believe to be reasonable assumptions. You will note throughout the use of phrases such as “for the purposes of this analysis” and the like. The specific results are therefore of necessity approximate. However the differences evident between my conclusions and those appearing in the Congressional Report are not due to these approximations but to basic differences in approach and to apparent disregard (or misunderstanding) of critical information presented to NASA in the White Papers presented in the NEO workshop conducted in Vail, Colorado in June 2006.
The attached analysis is my own and is not a B612 Foundation product. While many of the points I make in this analysis, perhaps most, are shared by colleagues both in and outside B612 Foundation, I take full responsibility for them. I feel certain that there are errors in detail which I have not caught, but the primary findings are not dependent on minor errors, but rather on larger issues which should be openly and widely debated. This issue can have very serious consequences for the future and I strongly believe that open debate and transparency in analysis is an obligation. I am therefore making this letter and analysis available to the wider NEO “community” and I encourage you to reconsider your policy with regard to the December 2006 Final Report which is the basis for the recently submitted Congressional Report.
I am at your service re clarifying or elaborating on any aspect of this analysis. And while I cannot speak directly for them I know that many members of the extended NEO community are ready and willing to join me in addressing these and other issues of concern with the NASA Congressional Report.
Sincerely,
Russell L. Schweickart
Chairman, B612 Foundation
Encl: Independent Analysis of Alternatives
Independent Analysis of Alternatives that could be employed to divert a NEO on a likely collision course with Earth
Summary Findings:
- There are three current NEO deflection concepts which I consider in this analysis to be essentially ready for development, testing and deployment if needed; the gravity tractor, the kinetic impactor, and nuclear explosion, in ascending order of total impulse available. I therefore limit this analysis to these available options.
- 98% of the pragmatic NEO threat can be diverted by the use of the gravity tractor and kinetic impactor concepts. I.e. 98% of the realistic threat posed by NEO impacts will require no more total impulse for diversion than is available using the combination of kinetic impactor and gravity tractor.
- The use of the sufficient capability of the kinetic impactor/gravity tractor combination is highly valued not simply because the nuclear explosion capability is excessive but because the precision of the resulting deflection provides full assurance to a concerned world public that the deflection has been successful and has not resulted in the possibility of a near-term return of the NEO.
- The statistical probability of having to resort to the use of nuclear explosives to divert a threatening NEO in the next 100 years is approximately 1 in 1000. Stated differently, the frequency at which a NEO requiring the use of nuclear explosives for diversion would otherwise impact the Earth is approximately 1 in 100,000 years. Nevertheless it is available should such an improbable need arise.
- The characteristics of the gravity tractor and kinetic impactor are such that they nicely compensate for each others’ limitations. Most importantly the combined use of the kinetic impact/gravity tractor provides not only the capability to divert over 98% of the threat but also the ability to state with confidence that a NEO once deflected will not be left on a trajectory with a near term return impact.
- With the exception of testing the nuclear explosion option all three concepts should be analyzed in detail. Consideration should be given in the future to flight testing the kinetic impactor/gravity tractor combination to validate the capability and provide confidence to the world public. The further development of deflection concepts not considered in this analysis should also be encouraged.
- Attention should be given to the importance of timely decision-making. If timely decisions are not made when a NEO threat arises the window of opportunity for the use of non-nuclear means for deflection can irrevocably be passed thereby leaving the world with the Hobson’s choice of using nuclear explosions in space for deflection or taking the chance of a devastating impact. This timing challenge applies even for the case of very small NEOs.
- Considerable information characterizing the nature of the NEO threat is available in the current Spaceguard Survey database and should be extracted from it to more fully understand both the frequency of resonant return impacts and the decision timing challenge in the light of episodic tracking opportunities.
Definitions, abbreviations, etc.
For the purpose of this analysis I will use the following terms for brevity.
Congressional report – The Near-Earth Object Survey and Deflection Analysis of Alternatives, Report to Congress., March 2007
Final report – 2006 Near-Earth Object Survey and Deflection Study, Final Report, Dec 2006 (internal distribution only, access denied to public and outside experts)
KI – kinetic impact or kinetic impactor; a deflection method utilizing the energy and momentum of a spacecraft directly impacting a NEO to achieve a change in its orbit.
GT – gravity (or gravitational) tractor; a deflection method utilizing the mutual gravitational attraction of a spacecraft “hovering” immediately in front of or behind a NEO to affect a desired change in its orbit.
Nuclear – any deflection method using a nuclear explosion to affect a desired change in the orbit of a NEO.
Beta – the multiplier of the KI impact momentum resulting from the ejecta of NEO material from the NEO surface. Based on data presented at the recent PDC07 (AIAA Planetary Defense Conference) I will conservatively assume the value range of 4 +/- 2 for this key variable.
Total impulse – the change in momentum required to deflect a NEO or the momentum change available from any given deflection method. (units; kg-m/s or Ns)
PoR – the Path of Risk. The narrow corridor across the face of the Earth within which a specific NEO will impact IF it does indeed collide with the planet.
PDC07 – The recent (5-8 Mar 07) AIAA Planetary Defense Conference held in Washington, DC
The world – this phrase is used as a proxy for the yet to be determined process by which nations will ultimately chose what action is appropriate in consideration of a NEO threatening an impact. As in “the world will not likely opt to “take the hit” for objects of Tunguska size.”
Pragmatic threat – that range of NEOs which the world will likely opt to deflect, assuming prior knowledge of a potential impact and the capability for deflecting it. This definition of NEO threat breaks strongly from the actuarial risk which is heavily biased toward the largest undiscovered NEOs. The pragmatic threat is characterized by the observation made at PDC07 that “a hit is a hit is a hit” vs. the actuarial measure of annualized lives lost globally. As pointed out by Alan Harris at PDC07, in an actuarial sense the annualized lives lost will always be dominated by that fractional large NEO that has not yet been discovered.
What deflection concepts are available and should be considered?
There are three concepts for deflecting NEOs which employ currently available technology and which require a minimum of additional analysis, development, and for the non-nuclear options, testing and demonstration. These three are, in ascending order of total impulse capability, the gravity tractor, kinetic impact, and nuclear explosions, and are the only three options dealt with in this analysis.
Several other deflection concepts have been put forward but these are not considered due either to the need for considerable advancement in technological capability or the requirement for specific, currently unavailable knowledge about NEOs themselves such as how attach securely to the surface of an asteroid. In this category are large space mirrors or powerful lasers for ablating NEO surface materials, the Asteroid Tugboat which attaches to and pushes directly on the asteroid, mass drivers in a variety of configurations which must also attach to the NEO and require considerable in situ construction, and suggestions of deflection by altering the coloration and thermal characteristics of the NEO surface.
Gravity Tractor Of the three concepts considered viable in the near term, the gravity tractor (GT) offers the least total impulse but imparts that limited impulse in a fully controlled manner. The gravity tractor (Nature, November 2005) must execute a full rendezvous with the NEO (velocity matching) and then actively “hover” either immediately in front of the NEO or behind it in order to either increase or decrease the NEO’s velocity respectively. Since a gravity tractor spacecraft, in any configuration, will carry aboard an active radio transponder its position and velocity are very well known before, during and following the deflection maneuver. These characteristics of the GT provide a dramatic improvement in the knowledge of the NEO orbit both prior to any deflection maneuver and, if a deflection is required and performed, precise knowledge of the resultant NEO orbit.
Of note is the fact that through the use of its transponder a GT, deployed to a NEO with a high probability of impact, will discover in many instances that there is no need for a deflection. In those instances where a deflection is indeed found to be required, either the GT itself can be used to provide the required deflection, or if the total impulse required is outside its capability, observe the deflection impulse provided by a kinetic impactor (KI) and subsequently determine precisely the result and even “trim” the outcome if necessary. This synergetic use of the GT and KI offers a very powerful combination.
Kinetic Impactor The KI imparts a desired change in a NEO’s orbit by crashing into it, in the desired direction and with a predetermined impact velocity and impactor mass. The change in velocity of the NEO is controlled by two factors; the momentum directly transferred to the NEO by the KI and the additional momentum provided by the mass and velocity of the NEO material ejected from the impact site per se. In many if not most cases the ejecta component of the momentum change exceeds that due directly to the momentum transferred directly by the KI. The ratio of the total momentum realized to the momentum of the KI at impact is expressed as the parameter beta, or the momentum multiplier.
The specific value of beta for any given impact is known very imprecisely. Recent work by Keith Holsapple and others indicates that beta increases with the energy of the impactor but is highly dependent on the specific characteristics of the particular NEO being impacted. For relative impact velocities typically between 5 and 15 km/s beta appears likely to have values no lower than 2 and not likely higher than 10. For the purposes of this analysis beta is considered to have a value of 4 +/- 2. Since beta is a very powerful influence on deflection performance and its maximum value could be much higher it should be a priority for further research, both analytic and (hopefully) empirical. In summary the total impulse imparted to the NEO for a KI is equal to beta x the impactor momentum. Given that the total impulse delivered to a NEO can vary by a factor of at least 3 the resultant orbit of the NEO is uncertain. This unavoidable uncertainty in the result of the deflection is the reason a KI (or nuclear) deflection is described here as uncontrolled.
There is, as was presented at PDC07, an opportunity for the use of a series of KI impacts should the total impulse required exceed that available in a single KI mission. Nevertheless the uncertainty in the resulting NEO orbit due to the variability in beta remains whether for a single or multiple impact deflection.
A further consideration of the KI employed alone, is that since it does not rendezvous with the NEO but rather simply crashes into it, it cannot determine whether or not, in fact, a deflection is required. For this reason the European Space Agency (ESA) in the development of its Don Quijote mission, has come to believe that a precursor rendezvous mission with a transponder is needed prior to the launch of an impactor, both to collapse the uncertainty in the NEO orbit (and therefore determine whether a deflection is in fact required) and to determine post-impact the resultant NEO orbit. ESA’s position is that a KI should not be used as a stand-alone but rather in combination with a precursor spacecraft which first rendezvous with the NEO and provides both pre- and post-impact precision tracking. I fully concur in this finding and see the KI as a viable deflection concept only in combination with a transponder-bearing spacecraft which plays this crucial pre- and post-impact role.
It is clear, based on the above, that the performance of the KI is considerably enhanced, both prior to and post impact, by the presence of a supporting transponder mission. Later in this analysis I show an even greater value in the KI/GT combination in the potential for the GT subsequent to the KI impact to not only determine the precise result of that impact but also to “trim” or slightly modify the deflection to insure that the NEO does not end up in an orbit with a resonant return impact within the next few decades.
Nuclear explosive The use of nuclear explosives for NEO deflection has been proposed in several forms ranging from subsurface emplacement to stand-off explosion. There are many technical unknowns about all of these configurations and I will not in this analysis attempt to delve into them. Suffice to say, for the purposes of this analysis, that while there are many unknown technical factors to be examined the nuclear explosives option offers a larger total impulse capability than any other available concept albeit with a very high uncertainty as to the momentum transfer achieved. Once again, as with the KI, the deflection is uncontrolled with considerable uncertainty in the post-deflection NEO orbit. Whether a stand-off GT could be used to serve both the pre- and post-deflection functions listed above for the KI would depend on it being able to survive the nuclear explosion itself.
The other obvious considerations re the nuclear option are the international legal prohibitions and the world-wide public concern with most things nuclear and especially weapons. The challenge of obtaining widespread international agreement that a nuclear explosion should be used in deflecting a NEO will be daunting, to understate it. Nevertheless, if the world is unable to come to agreement in time to utilize a non-nuclear deflection method the final option, due to its greater total impulse capability, will be nuclear. The ultimate alternative to using a nuclear device for diverting a NEO is “taking the hit”. This is an ominous choice given the psychological and physical implications of mass evacuations, refugees, destruction of infrastructure and the like. This reality should put strong emphasis on the world dealing early with the challenge of making timely NEO deflection decisions.
JUXTAPOSITION with NASA’s Congressional Report
The conclusions in NASA’s Congressional Report regarding the technological readiness and costs of a variety of deflection concepts appear to be seriously flawed. Without access to the Final Report however it is not possible to specifically examine the basis for these judgments. The primary differences between this analysis and NASA’s are the more limited set of deflection concepts considered (I consider only those which I believe will be available for use within the next several decades), and the extreme underestimate of the technological readiness level and equally extreme overestimate of the cost of the gravity tractor. While not available for detailed review without access to the Final Report, it appears from graphics shown briefly during PDC07 that the Report has erroneously equated the GT concept with the extremely expensive and technically immature Prometheus spacecraft. (NASA cancelled this program two years ago, largely for run-away cost reasons) The GT concept, as presented in NASA’s NEO Workshop held in Vail, Colorado in June-July 2006, is adaptable to any size spacecraft and was specifically presented and evaluated in White Paper 42 (the only professional paper presented on the gravity tractor) based on NASA’s Deep Space 1 spacecraft flown successfully from 1998-2000 and costing (based on the official NASA website, http://nmp.nasa.gov/ds1/quick_facts.html) $149.7 million (FY 95-99). Given that the presentations at NASA’s Vail Workshop explicitly presented and evaluated the GT concept based on the Deep Space 1 mission technology, and not on the cancelled Prometheus, it is mystifying why, in NASA’s Report to Congress the GT was presented as technically immature and extremely costly. Without access to the Final Report it cannot be stated with certainty but it appears that NASA inappropriately used the low technological readiness level and extreme cost of the cancelled Prometheus spacecraft as a proxy for the GT. In this analysis I use the GT as it was presented to the NASA study team in the NASA NEO workshop in Vail, Colorado.
What is to be deflected?
In order to analyze the efficacy of any NEO deflection method one must first determine or define the challenge being addressed. In this instance it is the range of total impulse required to deflect the NEOs most likely to trigger a deflection decision.
The bottom of this range is assumed herein to be a Tunguska-like NEO based on the assumption that nations, knowing that a Tunguska class object is threatening an impact, and knowing that the means to deflect it are available, will not sit idly by and “take the hit”. While the consequences of such an impact are, from an actuarial point of view, not likely to kill many people, the public presumption will be that the NEO will impact in the worst possible spot along the PoR where it crosses the territory of concern. For many of these small NEOs the PoR will not have resolved to a specific local area, let alone a point, by the time a decision to deflect it will have to be made. The image of Tunguska entering over London vs. Siberia will not be dispelled by probabilistic assurances of how unlikely it would be that any particular city would be located at “ground zero”.
From recent work by Mark Boslough of Sandia National Laboratory presented at PDC07 the most likely impact energy of the Tunguska asteroid is ~ 5 MT. His impressive simulations indicate that due to a finite “pancake” form (vs. a point source) of the NEO, and its downward momentum, past analysis of damage done on the ground at Tunguska has been overestimating the impact energy by a factor of 3 to 4. Further consultation with Alan Harris convinces me to use 5 MT as the current best estimate of the Tunguska impact energy.
Using Alan Harris’ most recent analysis of the size-frequency plot for NEOs we see (Fig 1) that a 5 MT impact energy equates to a NEO 45 meters in diameter with an impact frequency of just under 1 in 1000 years. The population of objects this size is estimated at 400,000 according to the most recent size-frequency plot.
[note: The best estimate of the size-frequency distribution of NEOs is judged (by Alan Harris and others) to be represented in Fig. 1 by the observations below the constant power law line (long blue dash) which Harris believes best represents the actual size-frequency distribution. For this reason I have conservatively chosen to use the “dip” vs. the constant power law distribution in this analysis. Were I to use the constant power law line the population of Tunguska-like NEOs would be over 1 million (vs. 400,000) and the frequency of impact 1 in 500 years (vs. 1 in 1000 years)]
With this defining the lower bound for the analysis of total impulse required, I then determine the upper bound of the most likely 99% of NEOs to be deflected by decreasing the population by two orders of magnitude (i.e. 4,000) which corresponds with a 400 meter diameter object with an impact energy of 3500 MT and an impact frequency of just under 1 in 100,000 years.
99% of the pragmatic threat then originates with objects between 45 and 400 meters in diameter, and it is heavily skewed toward the much larger numbers of the smallest objects.
JUXTAPOSITION with the NASA Congressional Report:
The Congressional report assumes a set of 7 challenging cases (1A through 6) which are highly improbable if one considers the most likely deflections to be called for. All but the Apophis 1A & 1B cases (addressed below) lie within the least likely 10% of potential NEO impacts that statistically threaten the world. My analysis determines the most likely 99% of NEOs to call for deflection and evaluates the deflection options against this challenge.
The Congressional Report presentation of the Apophis 1A and 1B scenarios is, on plain reading of the description, erroneous. It is likely that the Full Report goes into greater detail in the definition of these scenarios, but absent access to it no detailed critique can be made. However, in addressing the Apophis case de novo, it is an extremely moderate deflection challenge and lies well within the capability of the most modest GT. Furthermore, in employing a GT for such a deflection the uncertainty in the outcome of the deflection is very low due to the transponder availability on the GT. Therefore a deflection resulting in a 2036 Earth miss distance of 3 Earth radii (from the geocenter) is more than adequate to reach a collision probability in 2036 of less than e-6.
The selection of scenarios used in the NASA Congressional Report drive the conclusions. It would seem to go without question that if one selects for the evaluation scenarios exceptional challenges (challenges requiring exceptionally high total impulse) then the inevitable conclusion will be that nuclear explosions are the preferred response. Clearly this is circular reasoning and the basis for the selection of hypothetical scenarios must be examined. In this analysis I identify what I believe to be the minimum sized NEO likely to trigger a deflection decision and then extend the analysis to include the deflection of NEOs 100 times less frequent, i.e. the most likely 99% of deflections likely to be called for. Nuclear explosions may be needed in certain extreme and unlikely circumstances, but excessive force is not to be valued when more than adequate and far less controversial means are available. The nuclear option should be the choice of last resort.
What is the range of total impulse required for deflection of the pragmatic threat?
The lower bound is defined by the smallest NEOs of concern; those ~45 meters in diameter. The mass of such an object is roughly 130,000 metric tons. The total impulse required for deflection requires mass to be multiplied by the change in velocity needed to divert the object, and this number is highly variable, depending on the circumstances. In this portion of the analysis I will consider only the case of a direct impact with Earth, i.e. no intervening close gravitational encounter between the discovery of the threat and the nominal impact. The very powerful effect of such close gravitational passes on the velocity change required for deflection of a NEO will be dealt with separately below.
The velocity change required in a direct impact is strongly dependent on the time between the deflection maneuver and the nominal impact and somewhat weakly dependent on the particular orbit of the NEO. The goal of the Spaceguard Survey is to provide the world with “decades of early warning” of a pending impact and for the purposes of this analysis I will assume that a deflection will be executed 10-20 years prior to impact. Considering the change in velocity required for several known cases (Apophis and 2004 VD17) I will consider, for this analysis, the typical delta v required for each Earth radius of deflection to be 0.1 – 0.3 cm/s, and therefore use the average of 0.2 cm/s as representative.
The lower bound then on the total impulse for deflecting the smallest objects considered is approximately 2.6e5 kg-m/s per Earth radius of deflection. The comparable value for NEOs 100 times less likely to impact Earth, i.e., those ~400 meters in diameter is 1.8e8 kg-m/s. The remaining cohort of NEOs larger than 400 meters (i.e. less than 1 % of the pragmatic threat) are simply considered to require greater than this total impulse.
What is the range of total impulse available using the two non-nuclear deflection methods?
The GT performance is dependent on the mass of the GT spacecraft and the distance at which it hovers ahead of or behind the NEO center of mass. For the purposes of this analysis I will consider only a very modest GT based primarily on hardware already flown and tested, specifically the Deep Space 1 mission of 1998-2001. Assuming the spacecraft mass at 1 metric ton and hovering at 1.5 NEO radii above the center of mass of a 45 meter diameter NEO, I arrive at a total impulse applied of 658 kg-m/s per day, or a deflection period of 395 days to cause the NEO impact point to be displaced by 1 Earth radius. Since an actual deflection will need to be targeted to miss impacting the Earth by 3 or more Earth radii (see below), the minimum sized asteroid of concern would require a 1 metric ton GT to “tow” it for more than three years to accomplish a deflection. This is then a marginal case.
For a KI the calculation is simple but leaves one with a large uncertainty. A one metric ton KI with a relative velocity at impact of 15 km/s will impart a direct momentum transfer of 15e6 kg-m/s. Assuming a nominal beta of 4 this results in a nominal total impulse capability of 6e7 kg-m/s or a range of 3-9e7 kg-m/s. Translated this results in a more than adequate capability to deflect a 45 meter NEO or, at the upper end of the distribution the need for 6 KI impacts of this capability to deflect a 400 m NEO by 3 Earth radii assuming a minimum value of 2.0 for beta
I assume, albeit without any specific evidence or knowledge, that some form of the nuclear option may be able to divert a 400 meter object with a single explosion. In any event, whether single or multiple nuclear explosions are required the end result will be a priori highly uncertain. Furthermore whether or not this can be accomplished without fragmentation of the NEO is an unknown requiring further study.
Targetting considerations
A key question to be answered is what should the end target be when deflecting an asteroid? The worst case challenge is if the nominal impact point for the NEO of concern is located at the midpoint of the PoR.. the locus of potential impact points across the Earth. A deflection of 1 Earth radius, assuming the PoR lies near the equator would cause the deflected NEO to skim the Earth’s atmosphere.
Clearly this is an unacceptable lower bound for a deflection. More realistically one would want to deflect a threatening NEO to take it outside the Roche limit, the minimum distance at which the asteroid would not fragment into several pieces. While the calculation of the Roche limit is a complex one a value of 2.5 Earth radii from the geocenter is roughly correct. Therefore for the purposes of this analysis a reasonable minimum target for the NEO miss distance will be assumed to be 3 Earth radii from the geocenter.
Conclusion re NEOs on a direct impact course
For NEOs on a direct impact trajectory the GT is inappropriate for use with even the smallest NEOs (45 meters) in the pragmatic threat cohort. Were a GT to be used to deflect a 45 meter NEO to a distance of 3 Earth radii it would require a deflection duration of 1185 days or 3.25 years.
The KI method, using a single KI, would however be adequate for NEOs up to 220 meters in diameter using the nominal value of beta or objects of 155 meters diameter using the minimum value of 2. Multiple KI impacts could extend this capability.
In conclusion then while the GT is impractical for diverting any asteroids of concern on a direct impact trajectory with Earth, the KI can deflect objects up to 155 meters in diameter with a single impact and the minimum value of beta. If one considers multiple impacts it would require 3 in order to successfully deflect a 225 meter asteroid by 3 Earth radii with a beta of 2.0. Were a beta of 6.0 assumed a single KI impact could deflect a 225 meter NEO by 3 Earth radii or, with three impacts, a 325 meter NEO.
These performance numbers result in a single KI mission of 1 metric ton and relative velocity at impact of 15 km/s and a minimum beta value of 2 being able to deflect by 3 Earth radii 97% of the pragmatic NEO threat with a single impact or 98% with three impacts. If a NEO in the largest 2% of the pragmatic threat were to threaten an impact a nuclear explosive may have to be used.
JUXTAPOSITION with the NASA Congressional Report:
6 of the 7 “hypothetical scenarios” of NEO impacts used in NASA’s Congressional Report require greater total impulse for deflection than is available using the highest beta KI deflection. In this analysis, using the pragmatic threat cohort of NEOs all but approximately 1% of the threat can be deflected by use of a 1 metric ton KI with a relative impact velocity of 15 km/s.
How is this analysis affected by taking into account resonant return trajectories and associated keyholes?
This issue, dealt with specifically in White Paper #39 presented at NASA’s NEO workshop in Vail, has a very powerful influence, not only on the total impulse required to deflect an incoming NEO, but also on deflection targeting and the ability of a deflecting agency to assure the world that the deflection has been successful. For reasons not evident in the Congressional Report it appears that none of these influences were taken into account in the NASA analysis.
Resonant returns and their associated keyholes are defined by the NEO returning to the impact intersection an integral number of years later if it passes through the keyhole. E.g. for the asteroid Apophis, which will make a very close pass by the Earth on 13 April 2029 there is a keyhole close to its point of closest approach to the Earth such that if it passes within that very narrow region (in this case 600 meters wide) it will end up in an orbit with a period of 426.125 days and therefore return 7 years later (6 Apophis orbits around the Sun) and impact Earth.
If one looks at the NEOs with a non-zero probability of impacting Earth and travels backward in time from the potential impact one finds that in many cases there is a prior close pass by the Earth which sets the asteroid onto a course leading to the potential impact. The potential impact of Apophis in 2036 is such a case. In this instance if Apophis were to impact the Earth in April 2036 it would first have had to pass through the 7/6 keyhole as it passed the Earth in 2029.
The good news about keyholes is that if one deflects a threatening NEO prior to it passing through the keyhole the total impulse required to insure that it misses the Earth is reduced by orders of magnitude. Just how much lower the required total impulse is for deflection depends strongly on the specific orbital dynamics but it commonly ranges from 1 to 4 orders of magnitude and occasionally more, such as in the case of Apophis.
The effect of this is that for threatening NEOs that must pass through a keyhole prior to impact considerably less total impulse is required for deflection in order to avoid an impact, provided the deflection is performed prior to keyhole passage. E.g. while a 1 metric ton GT cannot deflect a small 45 meter diameter NEO on a direct impact trajectory in less than approximately 3 years, it can deflect the 280 meter Apophis in less than 40 days.
The relevant question then is what percentage of the population of Earth impactors will have first had to pass through a keyhole prior to impact, and how great a reduction in total impulse required for deflection is realized? Unfortunately the answer to this question is not known, though data mining of the current NEO database could provide us an excellent statistical answer. This research should be done but it is not on NASA’s work plan primarily because no one is currently funded to work on the issue of deflection in the NASA/JPL program.
What can be said however is that the incidence of close prior encounters with the Earth prior to impact (another way of saying passage through a keyhole) are not uncommon and may range up to 30% of cases or higher. Again, for the purposes of this analysis the fraction of these instances is assumed to be 30%.
Integrated into this analysis this factor reduces by 30% the number of NEOs which otherwise would require a nuclear explosion for deflection making them available for deflection by either the GT or KI. Indeed it further translates into a number somewhat below 30% of the entire pragmatic threat that are available to the GT alone, e.g. Apophis.
In summary the cohort of NEOs in the pragmatic threat category that can be deflected by non-nuclear means exceeds about 98% and many of them can be deflected by the GT alone.
JUXTAPOSITION with the NASA Congressional Report:
The NASA Congressional Report makes no specific mention of resonant returns or keyholes. The only implied recognition of their existence is found in “hypothetical scenario” A (Apophis) which uses the phrase “before its close approach to Earth in 2029” in describing the case. This in combination with the low total impulse required for Example A2 in Figures 4 and 5 leads one to the conclusion that this case assumes a predicted keyhole passage and a deflection prior to that event. The scenario then specifies however that Apophis must be deflected by 5 km to achieve a probability of collision less than e-6. The basis for this targeting goal (the only targeting addressed in the Report) is presumably contained within the NASA Final Report, but not available here for analysis. However, if taken as the deflection targeting miss distance (from the center of the 2029 keyhole) this requirement would result in a miss distance in 2036 of 17 Earth radii! Given that the Roche limit is at approximately 2.5 Earth radii and a very precise deflection of Apophis can easily be accomplished using a 1 metric ton GT (~a 40 day deflection maneuver), a deflection to 3 Earth radii is more than adequate. If, on the other hand, an uncontrolled deflection with a substantial uncertainty re the final Apophis orbit is assumed then, depending on the specific assumptions used the 17 Earth radii miss distance may be necessary.
In other words, if one assumes that the GT is not used for the A2 case and that it is not available to trim an uncontrolled deflection by either a KI or nuclear explosion, then the uncertainty in the result of the deflection may well require a very large targeted miss distance. However a very modest 1 metric ton GT can precisely deflect Apophis prior to its keyhole passage with a target of only 3 Earth radii. This is completely missed in the Congressional report.
Further implications of resonant returns and keyholes
One further consideration alluded to earlier is the issue of keyholes along the deflection path and their significance for uncontrolled deflections.
If one considers any deflection by a KI and assumes that the minimum value of beta is used to target the deflection so that the entire range of possible results lies at 3 Earth radii or greater, then clearly the outer limit of possibilities ranges out to 9 Earth radii. Along that very long path between 3 and 9 Earth radii lie thousands of resonant returns and keyholes through any one of which, albeit with low probability, the deflected NEO may pass. A significant percentage of these keyholes have uncomfortably short return times and, in the limit, can result in return impacts in less than 5 years.
Unless some means is employed to insure that a deflected NEO has not been “dropped” into one of these keyholes the world may find itself having avoided one impact only to have caused there to be another even more challenging impact by the same NEO. Despite the probability of this risk being fairly low (again, this can and should be specifically analyzed) without some means to positively control the deflection the best that can be said is “Yes, we deflected the NEO and it probably will not come back”. However, when dealing with the world public, especially after the anxiety surely to have accompanied the initial deflection, to not be able to assure positively that it was not dropped into yet another keyhole will certainly be difficult for the world to accept.
For this reason it is highly desireable that any uncontrolled deflection be immediately followed by a precise determination of the result and a means to adjust the deflection to avoid all keyholes if necessary.
Both of these conditions can be met with the combination of KI/GT. I.e. if a GT is always used as the precursor mission to a KI, then it is also in place to determine precisely (with its transponder) the actual result of the KI deflection and to determine whether or not the actual residual uncertainty includes a keyhole. In general it will not, but should this be the case then the GT can quite easily tow the asteroid slightly toward or away from the Earth in order to insure that there are no keyholes within the residual uncertainty of the deflection. In other words, the GT is there to both precisely determine the outcome of the KI deflection and, if necessary to trim it, thereby guaranteeing the world that the deflection will not result in a short term resonant return.
Thus the modest total impulse capability of the GT can easily compensate for the uncertainty in the KI deflection and the greater total impulse capability of the KI can be used with precision via the transponder and impulse trim capability of the GT.
JUXTAPOSITION with the NASA Congressional Report:
The NASA Congressional Report makes no mention of the possibility of a deflection placing the deflected NEO on a resonant return impact course. While the probability of such an eventuality is low it is by no means zero. The unavoidable consequence of an uncontrolled deflection is that the best that can be said, post deflection, is that the immediate impact has been avoided and that it is unlikely to return any time soon. With the use of a controlled deflection one will be able to provide positive assurance to an anxious world that there will be no short term resonant return.
In summary
On the order of 98% of the total pragmatic threat posed by NEOs impacting the Earth can be diverted from impact by the use of either the GT alone (for some resonant return NEOs) or the KI/GT combination. Only the least likely 1% of the threat (on the order of 1 in 100,000 years) will require the use of nuclear explosives for a successful deflection.
JUXTAPOSITION with the NASA Congressional Report:
Because of the “hypothetical scenarios” presented in the NASA Report to Congress the nuclear options are either best suited to the task or absolutely required. This selection of specialized scenarios is not, however, representative of the deflection cases most likely to be encountered. The highest probability impacts will always correlate with the smallest NEOs and, based on the assumption that the world will not choose to “take the hit” of a Tunguska-like object if its potential impact is known ahead of time, this cohort of NEOs is selected in this analysis as the lower limit of the pragmatic threat cohort. 99% of the NEOs in this cohort are more likely to call for deflection than those cases selected in the NASA Congressional Report. If those cases considered as examples require the use of nuclear explosions for deflection than it follows logically that the analysis will conclude that indeed nuclear explosives are required. There is no basis for arguing that excessive capability (i.e. nuclear explosion) is to be preferred over adequate capability, especially when the result of its application yields an uncertain and potentially dangerous result (the small but real risk of a short term return impact) and that there are many legal and social challenges to its use.
Russell L. Schweickart