FULL TEXT: NASA ESAS Final Report (DRAFT) October 2005: Section 13.0 Summary and Recommendations
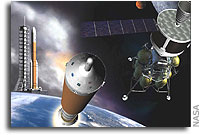
13. Summary and Recommendations
13.1 Lunar Architecture Recommendations
The lunar architecture defined by the Exploration Systems Architecture Study (ESAS) integrates mission mode analysis, flight element functionality and the activities to be performed on the lunar surface. An integrated analysis of mission performance, safety, reliability, and cost led to the selection of a preferred mission mode, the definition of functional and performance requirements for the vehicle set, and the definition of lunar surface operations. Additionally, the analysis looked back to examine how the Crew Exploration Vehicle (CEV) and Crew Launch Vehicle (CLV) would be used to transport crew and cargo to the International Space Station (ISS), and forward to define the systems that will carry explorers to Mars and beyond, in order to identify evolutionary functional, technological, and operational threads that bind these destinations together.
13.1.1 Mission Mode
The ESAS team recommends a combination of Earth Orbit Rendezvous (EOR) and Lunar Orbit Rendezvous (LOR) as the preferred lunar mission mode. The mission mode is the fundamental lunar architecture decision that defines where space flight elements come together and what functions each of these elements perform. The EOR-LOR mode is executed with a combination of the launch of separate crew and cargo vehicles, and by utilizing separate CEV and lander vehicles that rendezvous in lunar orbit. This mission mode combined superior performance with low Life-Cycle Cost (LCC) and highest crew safety and mission reliability. The lunar mission mode study initially considered a wide variety of locations of transportation “nodes” in both cislunar space and the vicinity of Earth. Initial analyses eliminated libration point staging and direct return mission options, leaving the mission mode analysis to investigate a matrix of low lunar (LOR) and Earth orbital (EOR) staging nodes.
13.1.2 Mission Sequence
The ESAS team recommends a mission sequence that uses a single launch of the Cargo Launch Vehicle (CaLV) to place the lunar lander and Earth Departure Stage (EDS) in Earth orbit. The launch of a CLV will follow and place the CEV and crew in Earth orbit, where the CEV and lander/EDS will rendezvous. The combination of the large cargo launch and the CLV is termed a “1.5-launch EOR-LOR” mission. Following rendezvous and checkout in LEO, the EDS will then inject the stack on a trans-lunar trajectory and be expended. The lander and CEV are captured into lunar orbit by the descent stage of the two-stage lander, and all four crew members transfer to the lander and descend to the surface, leaving the CEV operating autonomously in orbit. The lander features an airlock and the capability to support up to a 7-day surface sortie. Following the lunar surface mission, the lander’s ascent stage returns the crew to lunar orbit and docks with the orbiting CEV. The crew transfer back to the CEV and depart the Moon using the CEV Service Module (SM) propulsion system. The CEV then performs a direct-Earth-entry and parachutes to a land landing on the west coast of the United States.
13.1.3 Lunar Surface Activities
Recommended lunar surface activities will consist of a balance of lunar science, resource utilization, and “Mars-forward” technology and operational demonstrations. The architecture will initially enable sortie-class missions of up to 7 days duration with the entire crew of four residing and performing Extra-Vehicular Activities (EVAs) from the lunar lander.
The ESAS team recommends the deployment of a lunar outpost using the “incremental build” approach. Along with the crew, the lander can deliver 500 kg of payload to the surface, and up to 2,200 kg of additional payload if the maximum landed capacity is utilized. This capability opens the possibility of deploying an outpost incrementally by accumulating components delivered by sortie missions to a common location. This approach is more demanding than one that delivers larger cargo elements. In particular, the habitat, power system, pressurized rovers, and some resource utilization equipment will be challenging to divide and deploy in component pieces. The alternative to this incremental approach is to develop a dedicated cargo lander that can deliver large payloads of up to 21 mT.
The study team defined high-priority landing sites that were used to establish sortie mission performance. Of those sites, a south polar location was chosen as a reference outpost site in order to further investigate the operations at a permanent outpost. A photovoltaic power system was chosen as the baseline power system for the outpost.
13.1.4 Propulsion Choices and Delta-V Assignment
The ESAS team examined a wide variety of propulsion system types and potential Delta-V allocations for each architecture element. It is recommended that the CaLV’s upper stage will serve as the EDS for lunar missions and will perform the Trans-Lunar Injection (TLI) propulsive maneuver. The descent stage of the lunar lander was selected to perform Lunar Orbit Insertion (LOI) and up to 200 m/s of plane change using Liquid Oxygen (LOX)/hydrogen propulsion. The lunar lander descent stage will perform a coplanar descent to the surface using the same engine that performed LOI, and the crew will perform the surface mission while the CEV orbits autonomously. The lunar lander ascent stage will perform a coplanar ascent using LOX/methane propulsion that is common with the CEV SM propulsion system. The SM will perform up to a 90-degree plane change and Trans-Earth Injection (TEI) with full co-azimuth control (1,450 m/s total Delta-V).
Pump-fed LOX/hydrogen propulsion was selected for the lunar descent stage because of the great performance, cost, and risk leverage that was found when the lunar lander descent stage propulsion efficiency was increased by the use of a LOX/hydrogen system. To achieve a highreliability lunar ascent propulsion system, and to establish the linkage to in-situ propellant use, common pressure-fed LOX/methane engines were chosen for the CEV SM and lunar ascent stage propulsion systems.
13.1.5 Global Access
It is recommended that the lunar architecture preserve the option for full global landing site access for sortie or outpost missions. Landing at any site on the Moon sizes the magnitude of the LOI maneuver. A nominal 900-m/s LOI burn enables access to the equator and poles, and a maximum of 1,313 m/s is required for immediate access to any site on the lunar globe. The architecture uses a combination of orbital loiter and Delta-V to access any landing in order to balance additional propulsive requirements on the lander descent stage and additional orbital lifetime of the CEV systems. The lander descent stage was sized for a 900-m/s LOI plus a 200-m/s maximum nodal plane change, for a total of 1,100 m/s in addition to lunar descent propulsion. This value allows the crew to immediately access 84 percent of the lunar surface, and to have full global access with no more than 3 days loiter in lunar orbit.
13.1.6 Anytime Return
It is recommended that the architecture provide the capability to return to Earth in 5 days or less for sortie missions at any site on the lunar globe. The requirement to return anytime from the surface of the Moon to Earth was the design driver of the SM propulsion system. The lunar mission requires a total of 1,450 m/s of Delta-V, combining a 900-m/s TEI maneuver, a worst-case 90-degree nodal plane change, and Earth entry azimuth control. This capability enables “anytime return” if the lander is able to perform a coplanar ascent to the CEV. For sortie duration missions of 7 days or less, the CEV’s orbital inclination and node will be chosen to enable a coplanar ascent. Outpost missions will also have anytime return capability if the outpost is located at a polar or equatorial site. For other sites, loitering on the surface at the outpost may be required to enable ascent to the orbiting CEV.
13.1.7 Lunar Lander
The recommended lunar lander provides the capability to capture itself and the CEV into lunar orbit, to perform a plane change prior to descent, and to descend to the lunar surface with all four crewmembers using a throttleable LOX/hydrogen propulsion system. On the lunar surface, the lander serves as the crew’s habitat for the duration of the surface stay, and provides full airlock capability for EVA. Additionally, the lander carries a nominal payload of 500 kg and has the capability to deliver an additional 2,200 kg to the lunar surface. The lander’s ascent stage uses LOX/methane propulsion to carry the crew back into lunar orbit to rendezvous with the waiting CEV. The lander’s propulsion systems are chosen to make it compatible with In-Situ Resource-Utilization- (ISRU-) produced propellants and common with the CEV SM propulsion system.
13.1.8 ISS-Moon-Mars Connections
Evolutionary paths were established within the architecture to link near-term ISS crew and cargo delivery missions, human missions to the lunar surface, and farther-term human missions to Mars and beyond. The key paths that enable the architecture to evolve over time are the design of the CEV, the choice of CLV and CaLV, the selection of technologies (particularly propulsion technologies), and the operational procedures and systems that extend across the destinations. The CEV is sized to accommodate crew sizes up to the Mars complement of six. The CLV was chosen to be a reliable crew launch system that would be the starting point of a crew’s journey to the ISS, Moon, or Mars; and the CaLV was chosen, in part, to deliver 100-mTclass human Mars mission payloads to LEO. Propulsion choices were made to link propulsive elements for the purpose of risk reduction, and to enable the use of future ISRU-produced propellants. These propellant choices are further linked to the ISRU technology experiments to be performed on the planetary surfaces. Finally, EVA systems and mission operations will be developed to share common attributes across all ISS, lunar, and Mars destinations.
13.2 CEV Recommendations
One of the key requirements to enable a successful human space exploration program is the development and implementation of a vehicle capable of transporting and housing crew on Low Earth Orbit (LEO), lunar, and Mars missions. A major portion of the ESAS effort focused on the design and development of the CEV, the means by which the National Aeronautics and Space Administration (NASA) plans to accomplish these mission objectives. This section provides a summary of the findings and recommendations specific to the CEV.
It is recommended that the CEV incorporate a separate Crew Module (CM), SM, and Launch Abort System (LAS) arrangement similar to that of Apollo, and that these modules be capable of multiple functions to save costs. The CEV design was sized for lunar missions carrying a crew of four. Also, the vehicle was designed to be reconfigurable to accommodate up to six crew for ISS and future Mars mission scenarios. The CEV can transfer and return crew and cargo to the ISS and stay for 6 months in a quiescent state for emergency crew return. The lunar CEV design has direct applications to ISS missions without significant changes in the vehicle design. The lunar and ISS configurations share the same SM, but the ISS mission has much lower Delta-V requirements. Hence, the SM propellant tanks can be loaded with additional propellant for ISS missions to provide benefits in launch aborts, on-orbit phasing, and ISS reboost. Other vehicle block derivatives can deliver pressurized and unpressurized cargo to the ISS.
The ESAS CEV team’s next recommendation addresses the vehicle shape. Using an improved blunt-body capsule for the CM was found to be the least costly, fastest, and safest approach for bringing ISS and lunar missions to reality. The key benefits for a blunt-body configuration were found to be less weight, a more familiar aerodynamic design from human and robotic heritage (resulting in less design time and cost), acceptable ascent and entry ballistic abort load levels, crew seating orientation ideal for all loading events, and easier Launch Vehicle (LV) integration and entry controllability during off-nominal conditions. Improvements on the Apollo shape will offer better operational attributes, especially by increasing the Lift-to-Drag (L/D) ratio, improving Center of Gravity (CG) placement, potentially creating a monostable configuration, and employing a lower angle of attack for reduced sidewall heating.
A CM measuring 5.5 m in diameter was chosen to support the layout of six crew without stacking the crewmembers above or below each other. A crew tasking analysis also confirmed the feasibility of the selected vehicle volume. The recommended pressurized volume for the CM is approximately three times that of the Apollo command module. The available internal volume provides flexibility for future missions without the need for developing an expendable mission module. The vehicle scaling also considered the performance of the proposed CLV, which is a four-segment Solid Rocket Booster (SRB) with a single Space Shuttle Main Engine (SSME) upper stage. The CEV was scaled to maximize vehicle size while maintaining adequate performance margins on the CLV.
It is recommended that the CEV utilize an androgynous Low-Impact Docking System (LIDS) to mate with other exploration elements and to the ISS. This requires the CEV-to-ISS docking adapters to be LIDS-compatible. It is proposed that two new docking adapters replace the Pressurized Mating Adapter (PMA) and Androgynous Peripheral Attachment System (APAS) adapters on the ISS after Shuttle retirement.
An integrated pressure-fed LOX/methane Orbital Maneuvering System (OMS)/Reaction Control System (RCS) propulsion system is recommended for the SM. Selection of this propellant combination was based on performance and commonality with the ascent propulsion system on the Lunar Surface Access Module (LSAM). The risk associated with this type of propulsion for a lunar mission can be substantially reduced by developing the system early and flying it to the ISS. There is high risk in developing a LOX/methane propulsion system by 2011, but development schedules for this type of propulsion system have been studied and are in the range of hypergolic systems.
Studies were performed on the levels of radiation protection required for the CEV CM. Based on an aluminum cabin surrounded by bulk insulation and composite skin panels with a Thermal Protection System (TPS), no supplemental radiation protection is recommended. Solar arrays combined with rechargeable batteries were recommended for the SM due to the long mission durations dictated by some of the Design Reference Missions (DRMs). The ISS crew transfer mission and long-stay lunar outpost mission require the CEV to be on orbit for 6 to 9 months, which is problematic for fuel cell reactants.
The choice of a primary land-landing mode was primarily driven by a desire for land landing in the continental United States (CONUS) for ease and minimal cost of recovery, post-landing safety, and reusability of the spacecraft. However, it is recommended that the design of the CEV CM should incorporate both a water- and land-landing capability. Ascent aborts will require the ability to land in water, while other off-nominal conditions could lead the spacecraft to a land landing, even if not the primary intended mode. However, a vehicle designed for a primary land-landing mode can more easily be made into a primary water lander than the reverse situation. For these reasons, the study attempted to create a CONUS land-landing design from the outset, with the intention that a primary water lander would be a design offramp if the risk or development cost became too high.
In order for CEV entry trajectories from LEO and lunar return to use the same landing sites, it is recommended that NASA utilize skip-entry guidance on the lunar return trajectories. The skip-entry lunar return technique provides an approach for returning crew to a single CONUS landing site anytime during a lunar month. The Apollo-style direct-entry technique requires water or land recovery over a wide range of latitudes. The skip-entry includes an exoatmospheric correction maneuver at the apogee of the skip maneuver to remove dispersions accumulated during the skip maneuver. The flight profile is also standardized for all lunar return entry flights. Standardizing the entry flights permits targeting the same range-tolanding site trajectory for all return scenarios so that the crew and vehicle experience the same heating and loads during each flight. This does not include SM disposal considerations, which must be assessed on a case-by-case basis.
For emergencies, it is recommended that the CEV also include an LAS that will pull the CM away from the LV on the pad or during ascent. The LAS concept utilizes a 10-g tractor rocket attached to the front of the CM. The LAS is jettisoned from the launch stack shortly after second stage ignition. Launch aborts after LAS jettison are performed by using the SM propulsion system. Launch abort study results indicate a fairly robust abort capability for the CEV/CLV and a 51.6-degree-inclination ISS mission, given 1,200 m/s of Delta-V and a Thrust Weight (T/W) of at least 0.25. Abort landings in the mid-North Atlantic can be avoided by either an Abort-To-Orbit (ATO) or posigrade Trans-Atlantic-abort Landing (TAL) south of Ireland. Landings in the Middle East, the Alps, or elsewhere in Europe can be avoided by either an ATO or a retrograde TAL south of Ireland. For 28.5-degree-inclination lunar missions, abort landings in Africa can be avoided by either by an ATO or a retrograde TAL to the area between the Cape Verde islands and Africa. However, it appears that, even with 1,724 m/s of Delta-V, some abort landings could occur fairly distant from land. However, once the ballistic impact point crosses roughly 50-degrees west longitude, posigrade burns can move the abort landing area downrange near the Cape Verde islands.
13.3 Launch System Architecture Recommendations
The combined development of LV 13.1 and LV 27.3 pairs the most reliable, safest CLV with the most capable, most reliable, and highest performing CaLV. The development of the CLV based on LV 13.1 will provide the most straightforward, structured progression to the “1.5- launch solution” lunar architecture, while providing the lowest risk CLV development to acquire and maintain crewed access to LEO and the ISS. Using the existing fleet of expendable vehicles for scientific missions and robotic planetary missions allows the CLV/CaLV development to focus on providing a reliable, robust launch system to enable the national vision of continuing exploration of the Moon, and later on to Mars, as specified below.
13.3.1 Recommendation 1
The Nation should adopt and pursue a Shuttle-derived architecture as the next-generation launch system for 25-mT-class crewed flights into LEO and for 125-mT-class cargo flights for exploration beyond Earth orbit. After thorough analysis of multiple Evolved-Expendable- Launch-Vehicle- (EELV-) and Shuttle-derived options for crew and cargo transportation, Shuttle-derived options were found to have significant advantages with respect to cost, schedule, safety, and reliability. The Shuttle-derived options exhibited Loss-Of-Crew (LOC) values twice as safe as that of the EELV options and Loss-Of-Mission (LOM) values two to three times more reliable than those of the EELV options. Overall, the Shuttle-derived option was found to have the most affordable LCCs by leveraging proven vehicle and infrastructure elements and using those common elements in the heavy-lift CaLV as well as the CLV. Using elements that have a human-rated heritage, the CaLV can be human-rated to enable unprecedented mission flexibility and options by allowing a crew to potentially fly on either the CLV or CaLV for 1.5-launch or 2-launch lunar missions that allow for heavier masses to the lunar surface. The Shuttle-derived CLV provides lift capability with sufficient margin to accommodate CEV crew and cargo variant flights to ISS and potentially provides added services, such as station reboost.
The extensive flight and test databases of the Reusable Solid Rocket Booster (RSRB) and SSME give a solid foundation of well-understood main propulsion elements on which to anchor next-generation vehicle development and operation. The Shuttle-derived option allows the Nation to leverage extensive ground infrastructure investments to facilitate the future in space. Furthermore, the Shuttle-derived option displayed more versatile and straightforward growth paths to higher lift capability with fewer vehicle elements than other options.
The following specific recommendations are offered for LV development and utilization.
13.3.2 Recommendation 2
Initiate immediate development of a CLV utilizing a single four-segment RSRB first stage and a new upper stage using a single SSME. The reference configuration, designated LV 13.1 in this study, provides the payload capability to deliver a lunar CEV to low-inclination Earth orbits required by the exploration architectures and to deliver CEVs configured for crew and cargo transfer missions to the ISS. The existence and extensive operational history of humanrated Shuttle-derived elements reduce safety, programmatic, and technical risk to enable the most credible development path to meet the goal of providing crewed access to space by 2011. The series-burn configuration of LV 13.1 provides the crew with an unobstructed escape path from the vehicle using an LAS in the event of a contingency event from launch through Earth Orbit Insertion (EOI). Finally, a derivative cargo-only version of the CLV, designated in this report as LV 13.1S, can enable autonomous, reliable delivery of unpressurized cargo to ISS of the same payload class that the Shuttle presently provides.
13.3.3 Recommendation 3
To meet lunar and Mars exploration cargo requirements, begin development as soon as practical of an in-line Shuttle-derived CaLV configuration consisting of two five-segment RSRBs and a core vehicle with five aft-mounted SSMEs derived from the present External Tank (ET) and reconfigured to fly payload within a large forward-mounted aerodynamic shroud. The specific configuration is designated LV 27.3 in this report. This configuration provides superior performance to any side-mount Shuttle-derived concept and enables varied configuration options as the need arises. A crewed version is also potentially viable because of the extensive use of human-rated elements and other elements with human-rated heritage. The five-engine core and two-engine EDS provides sufficient capability to enable the “1.5-launch solution,” which requires one CLV and one CaLV flight per lunar mission—thus reducing the cost of each mission. The added lift capability of the five-SSME core allows the use of a variety of upper stage configurations, with potential growth paths to 120+ mT to LEO. LV 27.3 will require design, development, and certification of a five-segment RSRB and new core vehicle, but such efforts are facilitated by their historical heritage in flight-proven and well-characterized hardware. Full-scale design and development should begin as soon as possible after CLV development to facilitate the first crewed lunar exploration missions in the middle of the next decade.
13.3.4 Recommendation 4
To enable the 1.5-launch solution and potential vehicle growth paths as previously discussed, the Nation should undertake development of an EDS based on the same tank diameter as the cargo vehicle core. The specific configuration should be a suitable variant of the EDS concepts designated in this study as EDS S2x, depending on the further definition of the CEV and LSAM. Using common manufacturing facilities with the Shuttle-derived CaLV core stage will enable lower costs. The recommended EDS thrust requirements will require development of the J-2S, which is a derivative of the J-2 upper stage engine used in the Apollo/Saturn program, or another in-space high performance engine as future trades indicate. As with the Shuttle-derived elements, the design heritage of previously flight-proven hardware will be used to advantage with the J-2S. The SSME is not considered a viable candidate for powering the EDS due to its inability to be restarted without extensive preparation, which is essentially impossible to do during flight. The TLI capability of the EDS S2x is approximately 65 mT, when used in the 1.5- launch solution mode, and enables many of the CEV/LSAM concepts under consideration. In a single-launch mode, the S2B3 variant can deliver 54.6 mT to TLI, which slightly exceeds the TLI mass of Apollo 17, the last crewed mission to the Moon in 1972.
13.3.5 Recommendation 5
The Nation’s existing Expendable Launch Vehicle (ELV) fleet should be used to its fullest extent possible for launching lunar, planetary, and deep-space robotic probes in support of the exploration effort. The 5- to 20-mT lift capabilities available from ELVs provide a versatile set of vehicles applicable to a wide range of exploration mission requirements. ELVs possess highenergy upper stages with the capability to accelerate significant payloads to sufficient escape velocities for Earth-vicinity probes as well as deep-space vehicles, planetary orbiters, and landers for the outer solar system. In addition, the present fleet of expendables should be used to the maximum extent possible to deliver autonomous pressurized and unpressurized cargo and supplies to ISS. The ELV fleet is presently operational and requires no further development to undertake these missions for exploration or ISS support. Further, new commercially developed launch systems should be allowed to compete for these classes of missions as the new systems become available.
13.4 Technology Assessment Recommendations
As a result of the technology assessment, it is recommended that the overall funding of the Exploration Systems Mission Directorate (ESMD) for Research and Technology (R&T) be reduced by approximately 50 percent to provide sufficient funds to accelerate the development of the CEV to reduce the gap in U.S. human spaceflight after Shuttle retirement. This can be achieved by focusing the technology program only on those technologies required to enable the architecture elements as they are needed and because the recommended ESAS architecture does not require a significant level of technology development to accomplish the required missions. Prior to the ESAS, the planned technology development funding profile for ESMD was as shown in Figure 13-1. The ESAS recommendations for a revised, architecture-driven technology development is as shown in Figure 13-2.
Figures 13-3 through 13-5 show, respectively, the overall recommended R&T budget broken out by program with liens, functional need category, and mission. “Protected” programs include those protected from cuts due to statutory requirements or previous commitments.
The existing funding profile includes 10 percent management funds and approximately 30 percent of liens due to prior Agency agreements (e.g., Multi-User System and Support (MUSS), the Combustion Integrated Rack (CIR), and the Fluids Integrated Rack (FIR)) and legislated requirements (e.g., Small Business Innovation Research (SBIR), Small business Technology Transfer (STTR)). These liens include complicated questions about ESMD resources that are truly available to meet R&T needs. It should be noted that many of these items are not truly exploration-related research or technology developments and properly belong elsewhere. For example, MUSS is actually for ISS payload integration and should be in the Space Operations Missions Directorate (SOMD). Several of the recommendations below concern the realignment of budgets and functions in a more straightforward manner.
The final recommended technology funding profile was developed in coordination with the ESAS cost estimators using the results of the technology assessment. The following seven key recommendations arose from the technology assessment:
- ESMD should share costs with the SOMD for MUSS, CIR, and FIR. MUSS, CIR, and FIR are all ISS operations activities and, as such, should not be funded by ESMD R&T. Funds were identified in the recommended budget; however, cost-sharing plans should be implemented to ensure these facilities are efficiently operated.
- ESMD should transfer the Alpha Magnetic Spectrometer (AMS) to the Science Mission Directorate (SMD) to compete for funding with other science experiments. The AMS may be of scientific importance, but does directly contribute to meeting ESMD R&T needs. Therefore, it should be moved to SMD for consideration with other science missions.
- ESMD should quickly notify existing Exploration Systems Research and Technology (ESRT) projects not selected by ESAS that they will receive no funding beyond Fiscal Year 2005 (FY05). If work on the existing ESRT projects not selected for continuation is not stopped in FY05, there will be a potential for significant FY06 funds required to cover the contracts. Accordingly, appropriate notice must be provided as soon as possible to ensure efficient transition.
- ESMD should move Systems Analysis and Tool Development activities (and budget) to a directorate-level organization—no longer in ESRT. These system analysis and tool development functions should not be buried in multiple disparate organizations. While each organization will require its own analytical capabilities, a focal point should be established at the directorate level to ensure consistency in the ground rules, assumptions, and analytical methodologies across ESMD. This will ensure decision makers are provided “apples-to-apples” analysis results. These activities are also required to handle “whatif” studies and strategic analysis actions to provide greater stability in the development programs (i.e., development programs can focus on their work and avoid the disruption of frequent strategic studies and issue analyses).
- Key ESAS personnel should work with ESMD to facilitate implementation. Many technologies require immediate commencement on an accelerated schedule to meet aggressive development deadlines. Key ESAS personnel should also work with ESMD to ensure the analytical basis supporting ESAS recommendations is not lost, but rather carefully preserved and refined to improve future decisions.
- ESMD should develop a process for close coordination between architecture refinement studies and technology development projects. Technology projects should be reviewed with the flight element development programs on a frequent basis to ensure alignment and assess progress.
- ESMD should develop a process for transitioning matured technologies to flight element development programs. Experience shows that technologies have a difficult time being considered for incorporation into development projects due to uncertainty and perceived risk. The technologies identified in this assessment are essential for the architecture and, therefore, a structured process for transitioning them must be implemented to ensure timely integration into development projects with minimal risk and uncertainty. The key technology development project recommendations from the study are shown in Table 13-1.