Ed Lu’s Journal: Entry #8: Future Spaceships
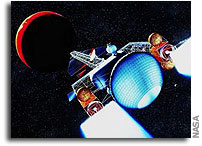
This
week I decided I’d write about some exciting new developments
that could really change the whole field of space flight.
The first has to do with getting off the planet cheaper, which
is the necessary first step that could truly make space flight
commonplace. The second has to do with getting from low Earth
orbit (where we are today) to further destinations like Mars,
the moon, and asteroids.
The place
to go find out about new and less expensive ways to get into
space is Oshkosh, Wisconsin, where the annual Experimental
Aircraft Association fly-in is being held this week. It is
a great place to see the latest in aviation – thousands of
aircraft and hundreds of thousands of people are at this moment
converging on Oshkosh. The theme of the show this year is
the Centennial of Flight – to mark the 100th anniversary of
the first powered airplane flight by the Wright Brothers at
Kitty Hawk.
Every
year, our friends Pete and Tom set up a campsite on the field.
We have a big group of aviation enthusiasts who fly in from
all over the place to camp out at what we refer to as Pete-n-Tom’s
Flights End CafÈ. This will be the first time in seven
years that I have missed going to Oshkosh, but I do have a
good excuse. My goal is to take a photograph of the thousands
of aircraft that will be parked on the airfield during the
airshow. I hope the weather cooperates.
Like
many of the astronauts, I got my start flying small general
aviation airplanes. One of my favorite things to do back home
is fly around in my little two-seat single-engine airplane.
My airplane is a kit-built airplane, as are many airplanes
nowadays. The fact that someone can go out and build their
own airplane, or go down to their local airport and take flying
lessons is what drives a lot of the innovation in aviation
today. Of course you can always just buy a ticket and fly
on an airline, too.
I hope
that someday flying in space becomes as commonplace as getting
on an airplane. Everybody should get a chance to see this
view!
Before
that can happen though, the cost and complexity of launching
things into space will have to come down. A lot of groups
and small companies are working on just that, and I think
that is a very good thing. None of these groups has actually
made an attempt yet at launching into space, so we’ll have
to see how this plays out.
One thing
for certain though is that there is a tremendous amount of
creativity and innovation out there, as anyone who has been
to Oshkosh can testify.
What
most of these groups are trying to do is build a ship that
can reach a speed of about 3,000 MPH, which is enough for
that ship to coast up out of the atmosphere to an altitude
of around 60 miles. Remember that to get into orbit around
the Earth, besides just getting out of the atmosphere, you
have to attain a speed of around 18,000 MPH. That means none
of these privately built ships is going to reach orbit at
first, but rather will coast up into space, and then gravity
will pull them back down to Earth – hopefully with a soft
landing. But at least they will be able to say they have been
to space, and they’ll get a quick taste of our view up here.
It’s a start. When private individuals and small companies
can reach space on their own, we will really start to see
space flight change our lives in the way that aviation has.
Once
you have gotten off the surface of the Earth, the next step
is getting out of orbit to further destinations like Mars,
the moon, and asteroids.
Here
is where the Space Station can really help us, as a test platform
for the two key technologies we need to really get out and
explore the solar system, power and propulsion. Propulsion
because everything depends on how fast you can go, and power
because something needs to run the engines you are using for
propulsion. Huge amounts of power will be required to conduct
science and research once you get to your destination.
The number
that tells you how fast a spacecraft can go is called the
“delta V” capability, which means the amount you
can change velocity using your own on-board fuel. The delta
V capability is all important because the bigger it is, the
more places you can reach and the faster you can get there.
To calculate the delta V capability of a spacecraft all you
have to know is what percentage of the mass of the ship is
fuel, and how fast the rocket engines spit the fuel out the
back.
For example,
on board the Space Station we have almost 4 tons of fuel,
which is used when we periodically fire small rocket engines
to boost our orbit higher as our orbit slowly decays (due
to the slight atmospheric drag). The Station has a mass of
about 200 tons, so that means a little bit less than 2 percent
of our mass is comprised of fuel. When we burn the fuel in
our rocket engines, the fuel becomes a gas that is ejected
out the back end of the engine at a speed of about -3.5 kilometers
per second, which provides the thrust to accelerate the Space
Station in the opposite direction (Newton’s laws again).
The faster
I eject the fuel and the more fuel I have, obviously the faster
I can go. I can demonstrate this law (known as conservation
of momentum) by floating in the middle of the module and throwing
some object (say a bag). I end up flying in the opposite direction
– but not at the same speed as the bag. If the bag is say
one-tenth my mass, then I will only react backwards at one-tenth
the speed of the bag. If the bag weighs as much as I do (we
have bags of water that are almost that big), then if I throw
that bag our resultant speeds are pretty closeÖ in fact
they would be identical if we had exactly the same mass. Think
of the bags as fuel, which is just something you throw backwards
to make yourself go forwards. If we were to burn all our fuel
on the Space Station, we would only be ejecting about 2 percent
of our total mass (the 4 tons of fuel), and therefore the
Space Station will only be accelerated by about 2 percent
of the speed of which we exhausted the gas, or in other words
about 70 meters per second (or about 150 MPH).
The 150
MPH we would gain would be a fairly small change in our total
speed (compared to our orbital speed of nearly 18,000 MPH)
and would boost our orbit only about 60 miles higher. What
this means is that the delta V capability of the Space Station
is sufficient to raise our orbit from 240 miles above the
surface of the Earth to about 300 miles. That means the Space
Station is pretty much going to stay in low Earth orbit, which
is where it was meant to be anyhow.
The delta
V capabilities of the Space Shuttle and the Soyuz are somewhat
larger, both being about 600 MPH. Of course, I am talking
here about how much additional speed they can attain after
they have already reached orbital speed and the main booster
rockets have burned out. The reason that these very different
looking ships have such similar capabilities is that both
were designed to be able to reach the altitude of an orbiting
Space Station and then return back to the ground. A 600 MPH
delta V capability is sufficient for that, but not much more.
So how
fast do we want to go?
In order
to fly to the moon, asteroids, or any of the other planets
in our solar system requires escaping the Earth’s gravitational
field. The speed it takes to do that depends on how far away
from the Earth you are, but very close to the Earth (as we
are) you need to be going about 25,000 MPH, which is about
7,000 MPH more than our orbital speed. So once you have reached
low Earth orbit, you need to be able to boost yourself by
at least another 7,000 MPH just to leave the vicinity of the
Earth.
That
means the Soyuz or the Space Shuttle, with a delta V capability
of about 600 MPH is over a factor of 10 less than the 7000
MPH needed.
The escape
velocity of 25,000 MPH is the bare minimum speed necessary
to really explore our solar system. Anything less than that
means you will remain in Earth orbit. And obviously, the faster
you can go the less time it takes to get to your destination
(which is important since the solar system is huge – our interplanetary
probes take months or years to get to their destinations).
If you
want to have people on these ships, it starts to get impractical
if the voyages take more than a few months in transit. Even
more delta V capability is necessary if you plan to actually
stop at your destination and return back to Earth.
Just
like flying across the module here – you first push off on
one side, coast across the module, and stop yourself on the
far side. If you want to return, you have to push off again
and fly back, and stop yourself again at your original point.
Every time a spacecraft has to use its rockets to speed up
or slow down, it uses up precious fuel. What this all means
is that a spacecraft that has a delta V capability, after
reaching orbit, of about 15,000 MPH is what is needed to really
start some exploration of our Solar System. So we are talking
about huge increases in performance since this is about a
factor of 25 more than our current capabilities.
We do
have small, unmanned spacecraft that have delta V capability
enough to get them a bit above escape velocity so we can send
them to other planets. These probes pick up almost all their
speed in the first few minutes after launch, then they coast
for the remaining months or years to get to their destinations.
That means we are currently able to send spacecraft to other
planets, but just barely. We have to wait until certain times
when the planets are properly aligned and resort to techniques
like “slingshotting” our probes from one planet
to the next to pick up additional speed.
This
situation is a bit like aviation 150 years ago, which at the
time consisted of ballooning. While ballooning was technically
flying, it really wasn’t useful as a mode of transportation
since you were at the mercy of the winds, and you couldn’t
carry much. If the wind was not blowing the way you wanted
to take your balloon, then you had to wait for another day.
The advent
of powered flight, i.e., airplanes, made aviation truly useful.
From that point on you could fly to your location regardless
of the winds, you could carry cargo, and fly more or less
whenever you wanted.
What
we must do if we want to really open up the solar system is
to find a way to get our spacecraft to go very much faster,
so we can fly where we want, when we want, and carry lots
of stuff. You can see that there are two ways to get more
delta V capability: either carry more fuel (it’s like carrying
more bags to throw); or find a way to exhaust your fuel at
a higher speed (like throwing the bags faster). The first
solution works up to a point since you can only carry so much
fuel. The second solution works provided you can find a way
to expel the fuel at a higher speed.
How much
fuel can you carry?
If you
keep putting larger and larger fuel tanks inside a spacecraft,
you have to remove something else of equal weight to make
room, and pretty soon the spaceship is almost all fuel. In
fact that is the situation with all current spacecraft, if
you count the booster rockets used to get them into space.
For example, the Space Shuttle and its boosters on the launch
pad weighs about 5 million pounds, of which only about 5 percent
is the actual Shuttle – most of the remaining 95 percent of
the liftoff weight is just fuel. The same is true of the Soyuz.
In fact most of the fuel is used just pushing around the rest
of the fuel we are carrying!
Think
of it this way, suppose I am throwing bags to “rocket-propel”
myself. If I am carrying one bag, which weighs as much as
me, and I throw it, I will gain speed equal to the speed at
which I threw the bag. What if I want to go twice as fast?
I need to throw another bag. But to do that I first need 2
additional bags to accelerate me together with the final bag
I wish to throw. How about 3 times as fast? Then I need 8
bags, first I throw 4 to accelerate myself and 3 bags, then
I throw 2 to accelerate myself and one bag, then I throw the
final bag. The number of bags, and thus the total mass of
me plus my fuel, is exponentially growing with how fast I
wish to fly.
So the
delta V capability of a rocket is pretty much limited to a
few times the speed at which you expel fuel since otherwise
the rocket would have to be enormous (and almost entirely
full of fuel), with a small fraction being actual useful cargo.
For those of you who have mortgages, it is exactly the same
formula as compound interest, and the situation is the same
as paying off a loan stretched out over too many years. At
first almost all of your payments are just covering the interest,
with very little going to capital. By the time you are finished
paying off the loan, the original amount you borrowed can
be a small percentage of what you paid back in total.
So we
are left with spitting out the rocket exhaust at higher velocity.
That turns out to be just a question of chemistry – how much
energy can be contained in the fuel. As long as your rocket
burns some kind of fuel, you won’t get much more than about
12,000 MPH for an exhaust velocity, with more typical values
around 10,000 MPH. If you want to get 15,000 MPH out of a
ship, you will be a flying gas tank, and the size of the actual
useful part of the ship will be tiny (a few tons at most).
That’s one of the major challenges in sending a manned spacecraft
to other planets – they have to be large enough to accommodate
humans and all the equipment needed to keep them alive.
Luckily,
there is another way – instead of burning the fuel, we can
use electrical energy to accelerate it out the back of the
rocket.
These
types of engines are known as plasma or ion engines, and have
exhaust velocities as much as 20 times greater than any chemically-powered
rocket. Of course that comes with a price, they use a lot
of electricity. To date, these engines are quite small, and
don’t have a lot of thrust, but they get great gas mileage.
In fact, none of currently tested engines has thrust greater
than a few ounces! While that isn’t much, they use fuel at
such a low rate that they can run continuously for many months.
Since these engines have such tiny thrust, they are of no
use for lifting something off the ground into orbit. That’s
why conventional rockets are still important.
Once
in orbit the situation is different. The ship is now weightless
and you can take your time and gradually accelerate the spacecraft
to high speed. It’s the classic case of the tortoise beating
the hare. Up here when moving very heavy objects by hand we
use the same principle – rather than pushing very hard, you
just apply a very light touch for a longer time and you can
move objects weighing hundreds of pounds.
We have
already flown a spacecraft called Deep Space 1 that used an
ion engine to rendezvous with a comet. There are a number
of research groups working on making these engines much larger
so they will be practical for much larger spacecraft.
So once
you have a big plasma engine, how do you power it? Up here
on the Space Station we use solar cells for our power, but
they are big and bulky (when finished, our truss-work supporting
the arrays will be the size of a football field). And, of
course, the further away from the sun you travel, the less
light you have and the less power you get from the solar cells.
The amount of power that would be needed is hundreds of kilowatts
for smaller unmanned spacecraft, and many megawatts for larger
manned ships. Even the huge solar arrays of the Space Station
couldn’t come close to providing that much power.
So that
means you need a nuclear reactor to provide electricity for
your rocket engine. These reactors would be very small by
comparison with power plant reactors, and quite a bit simpler.
The nice part is that the reactor fuel can be launched “cold”
– meaning essentially non-radioactive. The fuel can then be
activated in the reactor once off the planet. This way if
there is a launch accident there is no risk of spilling radioactive
material, since the fuel has not yet been activated. As an
added bonus, by having a reactor on board you also have plenty
of power to run all the instruments and systems on the spacecraft.
Again there are several groups working to perfect the design
for small nuclear reactors for use on spacecraft.
The combination
of a nuclear reactor plus a plasma engine could provide a
delta V capability of 30 to 100 thousand MPH. This is plenty
for exploring the solar system.
The exciting
part is that NASA has decided to start just such a program,
known as Project Prometheus. The first proposed test of the
system is to fly a probe to Jupiter.
At first
these nuclear-electric propulsion systems will be only large
enough to power unmanned spacecraft, but if we are successful,
then in the future we should be able to scale them up to large
enough systems to carry people.
My feeling
is that the Space Station is an ideal place to develop and
test many of the components before we try them for real on
a deep space mission.
Probably
the two biggest unknowns are how to convert the heat produced
by the reactor into electricity, and how to make ion and plasma
engines both larger and run longer. The Space Station could
be the experimental laboratory for the development of these
crucial technologies. We could use a large solar reflector
to concentrate sunlight to test our system of converting heat
to electricity. The power generated could power a small plasma
engine which continually (but with small thrust) boosts the
Space Station to compensate for the small amount of atmospheric
drag.
In this
way, by incrementally testing each of the components of the
final system we will in the end, we will have much greater
confidence that it will work the way we expect once we put
all the pieces together.
Kitty
Hawk – where it all started 100 years ago.
The airfield
at Oshkosh – the future of aviation – where the Experimental
Aircraft Association fly-in is being held. I can see Pete
and Tom’s campsite from here!