Ringing Protons Give Insight Into The Early Universe
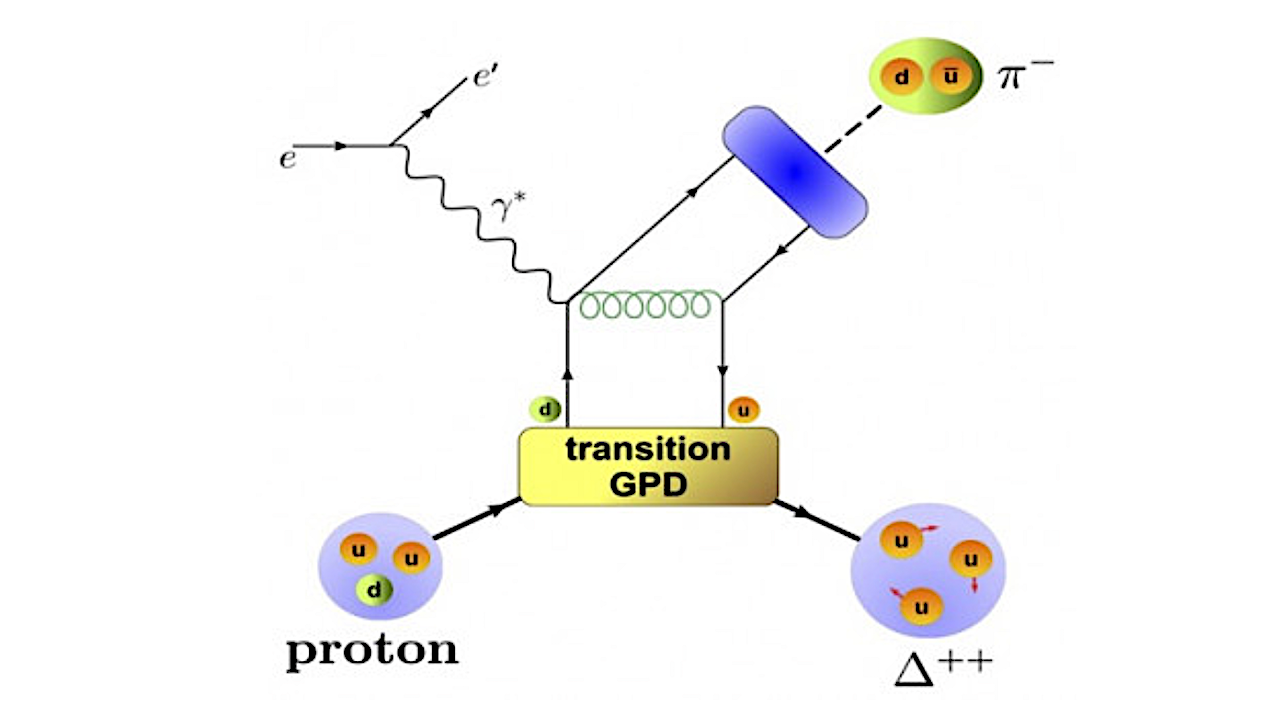
In the middle of the last century, physicists found that protons can resonate, much like a ringing bell. Advances over the last three decades have led to 3D pictures of the proton and significant insight into its structure in its ground state. But little is known about the 3D structure of the resonating proton.
Now, an experiment to explore the 3D structures of resonances of protons and neutrons at the U.S. Department of Energy’s Thomas Jefferson National Accelerator Facility has added one more puzzle piece to the vast picture of the chaotic, nascent universe that existed just after the Big Bang.
Studying the fundamental properties and behaviors of nucleons offers critical insights into the basic building blocks of matter. Nucleons are the protons and neutrons that make up the nuclei of atoms. Each nucleon consists of three quarks tightly bound together by gluons by the strong interaction — the strongest force in nature.
The most stable, lowest-energy state of a nucleon is called its ground state. But when a nucleon is forcibly excited into a higher-energy state, its quarks rotate and vibrate against each other, exhibiting what’s known as a nucleon resonance.
A group of physicists from Justus Liebig Universitat (JLU) Giessen in Germany and the University of Connecticut led the CLAS Collaboration effort to conduct an experiment exploring these nucleon resonances. The experiment was carried out at Jefferson Lab’s world-class Continuous Electron Beam Accelerator Facility (CEBAF). CEBAF is a DOE Office of Science user facility that supports the research of more than 1,800 nuclear physicists worldwide. Results of the research were published in the prestigious peer-reviewed journal Physical Review Letters.
Analysis leader Stefan Diehl said the team’s work sheds light on the basic properties of nucleon resonances. Diehl, is a postdoctoral researcher and project leader at the 2nd Physics Institute at JLU Giessen and a research professor at the University of Connecticut. He said the work is also inspiring fresh investigations of the 3D structure of the resonating proton and the excitation process.
“This is the first time we have some measurement, some observation, which is sensitive to the 3D characteristics of such an excited state,” said Diehl. “In principle, this is just the beginning, and this measurement is opening a new field of research.”
The mystery of how matter formed
The experiment was conducted in Experimental Hall B in 2018-2019 using Jefferson Lab’s CLAS12 detector. A high-energy electron beam was sent into a chamber of cooled hydrogen gas. The electrons impacted the target’s protons to excite the quarks within and produce nucleon resonance in combination with a quark-antiquark state — a so-called meson.
The excitations are fleeting, but they leave behind evidence of their existence in the form of new particles that are made from the excited particles’ energy as it fritters away. These new particles live long enough for the detector to pick them up, so the team could reconstruct the resonance.
Diehl and others will discuss their results as part of a joint workshop on “Exploring resonance structure with transition GPDs” August 21-25 in Trento, Italy. The research has already inspired two theory groups to publish papers on the work.
The team also plans more experiments at Jefferson Lab using different targets and polarizations. By scattering electrons from polarized protons, they can access different characteristics of the scattering process. In addition, the study of similar processes, such as the production of a resonance in combination with an energetic photon, can provide further important information.
Through such experiments, Diehl said, physicists can tease out the properties of the early cosmos after the Big Bang.
“In the beginning, the early cosmos only had some plasma consisting of quarks and gluons, which were all spinning around because the energy was so high,” said Diehl. “Then, at some point, matter started to form, and the first things that formed were the excited nucleon states. When the universe expanded further, it cooled down and the ground state nucleons manifested.
“With these studies, we can learn about the characteristics of these resonances. And this will tell us things about how matter was formed in the universe and why the universe exists in its present form.”
Born in Lich, Germany, Diehl pursued physics as a means to understand the phenomena of nature and the nature of the world. He earned bachelor’s, master’s and doctoral degrees at JLU Giessen. He is a member of the CLAS, PANDA, ePIC and COMPASS collaborations and has co-authored more than 70 peer-reviewed publications.