Former Astronaut Don Peterson’s Ideas for Future Human Flight Programs
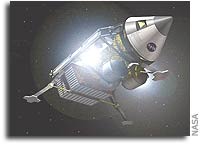
1. Future NASA Human Space Flight Programs Should Use a Modular Approach (Analogous to the current ISS configuration but with more configuration flexibility.)
The term “Modular Approach” means various functional requirements would be provided by individual, physically separate modules with standardized interfaces such that they could be assembled (stacked or clustered) in different combinations for launch or launched separately and joined on orbit (which implies they would have to be able to rendezvous and dock with other modules or be handled by a “space tug” vehicle) to create a spacecraft for a particular mission. Each module would provide the accommodations, tools, and capabilities to perform a specific function or group of related functions; for example:
- Crew Carrier Module for launch, abort, reentry, rendezvous, docking, etc. that would provide life support, communications, and other needed functions to support those operations
- Habitation Module with provisions for life support, hygiene, galley, medical, exercise, recreation, sleep, and other crew support functions for long duration orbital stay times
- On-Orbit Work Module with grappling/capture mechanisms, Airlock/EVA systems, a large work bay, lighting, data processing, etc. for on-orbit assembly, capture/repair, etc. missions
- Power/Conditioning Module with energy sources (Solar, Fuel Cell, Nuclear, or other), power conditioning and distribution systems, radiators and coolant provisions, etc. to provide power and conditioning to other modules.
- Tanker Module with tanks, pumps, valves, etc. to transport and store propellants, coolants, hydraulic, and other fluids as needed by other modules.
- Cargo Launch/Entry Module with appropriate restraints and deployment mechanisms for launch and recovery of large items. Could use parachutes and retro rockets (larger versions of the retros used for the Mars robot landers) for soft landing. Would not require human rating.
- Orbital Transfer Module (or Propulsion Module) with propulsion, attitude control, GN&C, capture/latching/docking mechanisms, etc. to move modules/cargo from one orbit to another (The ESA version might fill this requirement if configured with standard interfaces)
- Science Laboratory Module with interfaces for power and thermal conditioning, instrumentation, work stations (racks), etc. to support on orbit science operations (like SpaceHab)
- Others that I have not thought about.
Benefits include:
- Safety and Survivability. Redundancy of critical functions in two or more physically separate orbital modules offers protection against single destructive events such as fire, explosion, internal contamination, meteoroid impact, etc. Implementation would require modules be connected by two or more paths so that loss of a module would not isolate part of a space vehicle.
- Programmatic and Operational Flexibility. Different combinations of modules could simultaneously support different programs/missions, including: the Human- Mars Exploration program (HMEP – my acronym, not NASA’s), the ISS, Satellite Deploy and Retrieve/Repair/Return Missions, Science Laboratory Missions (a la SpaceHab), etc. In other words, we wouldn’t have to shut down everything that we previously told America was very important, in order to initiate HMEP.
- Infrastructure Creation. The set of modules as a whole would constitute an array of standard, proven, building blocks to support future programs and goals or deal with unforeseen contingencies in ongoing programs (e.g., replacement of a failed or damaged module in an orbiting configuration). New programs would not have to start from scratch and potential users could design equipment and plan activities based on well defined interfaces and reliable hardware and systems. Evolutionary changes could be made without disrupting entire programs.
- Political/Contractual Appeal. The work on various modules could be distributed among different NASA Centers and different contractors in different Congressional districts and even different countries. Work could be broken into modular chunks that could be “bought by the yard,” whenever funding was available. This may not appeal to some purists, but it sure helps with public, Administration, and Congressional support.
Drawbacks include:
- Non-optimal Performance. Modular configurations would be designed as generic building blocks for multiple missions and programs. By contrast, the Apollo and Shuttle designs were driven by the need to create the lightest weight, smallest, lowest power – and for Shuttle, least cost – combination of elements that would satisfy the performance requirements for their specific mission. To meet these demands, they had to be so tightly integrated that their components and subsystems were an interlocked set that are not suitable as building blocks in other programs. Confederated modules are highly unlikely to be optimal for any single mission from a weight, volume, power, or cost standpoint.
- System Integration Complexity. To make a modular approach work, there would have to be well-defined interfaces and standards (e.g., hatch sizes, grappling/docking fixtures; internal cleanliness; electrical power commonality; standardized display and control layouts, compatible software algorithms, and the list goes on…) rigidly enforced on all participants. Modular building blocks must fit together physically, work together functionally, and exhibit common operational features to facilitate crew training and minimize crew and ground control errors during operation, especially in time critical situations.
2. Do we really need to go back to the moon as part of a Human Mars Exploration Program (HMEP – my acronym, not NASA’s)?
An article titled “Reconnaissance,” on page 32 of the March 22nd ’04 issue of Aviation Week says, “NASA’s new lunar exploration program will start modestly with a $300-million orbiter using recycled planetary instruments to scout a landing site for future humans, but experts believe it could evolve into a surface complex that private industry would operate after NASA moves on to Mars.” The article focuses on the things that must be done to explore the moon, but does not discuss any specific lunar activities associated with the HMEP
However, statements by NASA spokespersons in the past few months indicate that NASA believes: (1) Mars vehicles could be launched from the moon to take advantage of the increased performance afforded by reduced gravity, (2) conditions on the lunar surface (about 1/6 earth gravity, no atmosphere, etc.) would serve as an ideal environment to develop and test equipment and procedures and train crews for operations on the surface of Mars (about 2/5 earth gravity, very thin atmosphere, etc.), and (3) operations on the moon would provide a relatively economical and safe “stepping stone” to Mars.
Each of the NASA statements raises some questions which are listed and briefly discussed in the following three subsections.
2.1 Mars vehicles could be launched from the moon to take advantage of the increased performance afforded by reduced gravity
Is it really more efficient and effective to launch the Mars vehicle from the moon? (I must be missing something!) First, everything that is to be launched from the moon will first have to be launched from the earth. Then it will have to be soft-landed on the moon. Next it will have to be assembled, fueled, and processed for launch. So not only will the entire Mars vehicle have to be launched from the earth; it will also be necessary to launch all the fuel and all the equipment to construct and maintain a facility on the moon that will be a kind of miniaturized lunar Kennedy Space Center with assembly buildings, gantry, launch control room and all the associated accoutrements (instrumentation, communication, cranes, hoists, tools, transporters, etc.) and a sizable work force with living quarters, galley, medical facilities, etc. to service the vehicle and maintain the facilities.
And lunar operations will be cumbersome. All facilities will have to be pressurized, shielded against radiation; and protected from dust. (Lunar dust was a serious problem during Apollo.) Workers will either have to wear pressure suits or travel from one pressurized building to another via airtight tunnels or vehicles. Extreme temperatures will occur during the long daylight and dark periods.
I believe what advantage there might be in launching the Mars vehicle in the lower gravity of the moon, will certainly not offset the additional cost, complexity, and risk involved in establishing and operating a lunar launch site.
If the Mars vehicle is too large to launch directly from the earth (which it probably is), a better alternative might be to assemble the Mars vehicle in orbit and launch form there (even less gravity than the moon). Of course to do this NASA would need a modular Mars vehicle design (see Item 1 above), a vehicle with Shuttle-like capabilities to enable equipment handling and EVA, and an orbiting assembly base, kind of like the ISS. (But NASA has apparently already decided we don’t need those kinds of capabilities in our future human space program.)
2.2 Conditions on the lunar surface would serve as an ideal environment to develop and test equipment and procedures and train crews for operations on the surface of Mars.
I’m sure that proponents of lunar testing and training are aware that the lunar environment differs significantly from the Mars environment as indicated by the examples in Table 1. So this NASA statement raises several questions about the value and validity of testing equipment and training crews on the moon.
2.2.1 Is lunar testing the most efficient and effective way to test various Mars Program test objects (i.e., objects intended for use in the HMEP, such as: rovers, pressure suits, solar arrays, radiators, batteries, habitats, communications antennae, etc.)?
Clearly, there are some aspects of the Mars vehicles and systems, such as aerobraking and parachute descent, that cannot be tested on the moon. Tests of some other systems and components, (e.g., solar arrays, energy storage devices, thermal conditioning systems, etc.) could probably be done with greater validity (and at much lower cost) in environmental chambers where the solar constant, day/night cycle, atmosphere effects, and temperature could model Martian conditions quite accurately.
In fact, it seems that the only truly unique factor in lunar testing is the effect of 1/6 gravity, which is important for systems and components that involve mass dynamics (e.g., rover vehicles, fluid flow devices, etc.). Even in these cases it isn’t obvious that testing devices designed for Mars gravity (about 2/5 earth) in lunar gravity (about 1/6 earth) will yield any more valid results than testing in earth gravity (again at much lower cost) using the same “reduced gravity emulators,” (underwater neutral buoyancy, sling supports and slanted walls to reduce weight effects, Zero-G aircraft, air bearing floors, etc.) that apparently produced satisfactory results in the Apollo Program. It is also worth noting that the robotic vehicles now on Mars were all successfully designed and tested without benefit of a low gravity test environment.
In any event, the differences between the lunar environment and the Mars environment will impose significant differences between the design and operation of lunar test objects (rovers, solar arrays, energy storage devices, thermal conditioning systems, etc.) and the analogous Mars objects. This in turn will necessitate some rather complicated manipulation of “modeling factors” to make lunar test results applicable to Mars equipment, and the program will incur extra cost and complexity to field and operate and analyze two sets of substantially different equipment in two significantly different environments.
2.2.2 Is it necessary to have humans in close proximity to a test site?
Traditionally human proximity to test sites has been extremely beneficial. Visit any active test site and you will see dozens of technicians setting up and checking out test equipment and objects before the test (positioning instrumentation and cameras; verifying wiring continuity; servicing batteries, coolant tanks, fire extinguishers, and other items with expendables or limited shelf life; etc.) and visually observing test results and inspecting test objects for necessary modifications and repairs after the test. And of course some tests involve dynamic “operator-in-the-loop” human factors evaluations. There is no question that these human contributions are important.
However, to be really effective, these activities usually require relatively large numbers of technicians with varied skills, specialized equipment and tools, work areas with electrical power, machine shops, abundant spare parts and supplies of expendables, transporters, hoists, and other equipment equipment-handing aids, etc. They cannot be adequately carried out by a few Martian-astronaut-trainees with hand tools. To support lunar testing, NASA will be required to provide multiple specialists and copious resources on the moon.
I think an effective lunar test program would require a much larger effort than the original Apollo Program; and so I think it would be much more feasible to design lunar test equipment and objects and develop test procedures and methods for robotic/remote operation (with enough redundancy to obviate the need for manual installation of spare parts) with little or no human on-site support.
2.2.3 Could we test Mars objects on Mars?
If test equipment and objects can be designed to operate entirely without human on site support, would it become more effective and efficient to test Mars objects on Mars? There are already vehicles and systems operating on Mars, and during the next decade there will likely be more of them. Most of them transmit performance and health data back to earth. Wouldn’t it be more effective and efficient (I almost said “cheaper, faster, better”) to use the data from these vehicles and systems to design early versions of the precursor vehicles and systems (including landers, communication, navigation, power, habitat, etc.) that we will send to Mars in preparation for human visits. Then the data from the precursors, operating in the real Martian environment, could be used to enhance the design of the final versions of the vehicles and systems to support human exploration of Mars. If testing on Mars is feasible, it would save a lot of money that will otherwise be spent on lunar operations.
2.2.4 Is it necessary (or of great benefit) that Mars astronauts undergo familiarization and training in reduced gravity on the moon?
I would defer to the Apollo folks who actually worked on the moon to assess the necessity and/or benefits of training in 1/6 earth gravity on the moon to prepare for work in the 2/5 earth gravity of Mars. However, it is worth noting that the Apollo astronauts were all trained in various earth-bound “reduced gravity simulators” and that was apparently sufficient to enable them to adapt quickly and safely and work very effectively in the moon’s reduced gravity. They even mastered the dynamic “operator-in-the-loop” task of “rover driving” by practicing on the earth with a 1-G version of the lunar rover. (Perhaps they were unusually adaptable people.)
3. Operations on the moon would provide a relatively economical and safe “stepping stone” to Mars.
Going to the moon is cheaper and safer than going to Mars. However, that’s not the question. The question is does it provide an economical stepping stone to Mars?
And I believe that using the moon as a “stepping stone” to Mars is neither very economical nor very safe
3.1 Cost. I have not seen any NASA Mars Program cost estimates other than the general statements about their annual budget, but I have a couple of ball park figures from the original Apollo Program. A pamphlet titled “The Early Years: Mercury to Apollo-Soyuz,” PM 001 (KSC), November 1985, which is part of a series titled “NASA Information Summaries” states (page 7) that the Apollo program costs were approximately $25 billion. An on-line article titled “Apollo program” from the “Wikipedia free encyclopedia” gives a figure of $25.4 billion. I assume these figures were obtained by simply adding up the reported NASA budget figures without any correction for inflation in the three plus decades since the program ended.
I then used the Inflation Calculator on the Bureau of Labor Statistics web site and found that inflation from 1973 (which I used as the end of the Apollo era) to the end of 2003 would make the Apollo Program cost $103.5 billion in “today’s dollars.” Harrison Schmitt has said it should not cost too much to reproduce the Apollo Program capabilities today. His article, titled “Figuring a New Way to Return to the Moon” on page 70 of the March 15, 2004 issue of Aviation Week; states “Developers of a new Saturn-based vehicle will know exactly what specifications need to be met and what design and operational approaches have worked and not worked.”
I don’t want to start a fight with Jack, but according to NASA, the Apollo blueprints and manufacturing jigs and fixtures have been lost. And most of the people who collectively preserved the knowledge of operational methods in their heads are no longer available.
More importantly, NASA’s announced lunar goals are considerably more ambitious than the original Apollo Program goals. The original Apollo program consisted of a total of eleven manned flights, nine of which (including Apollo 13) actually reached the moon, and six of which landed carrying a total of twelve astronauts to the lunar surface. The longest lunar surface activity (EVA) on any one mission was less than 23 hours (in three sorties) and each vehicle carried only a few hundred pounds of scientific equipment to the lunar surface.
By contrast, NASA is apparently proposing testing Mars equipment (rovers, habitats, etc.) on the moon and training Mars astronauts there. My guesstimate is that a Mars testing/training lunar program would require more than an order of magnitude increase in the amount of material and at least a five-fold increase in the number of people delivered to the lunar surface, with significant increases in stay time and facilities requirements (power, instrumentation, communication, etc.). The program will require new systems and new techniques, and even allowing for improvements in technology and our base of knowledge of lunar operations, it is likely that such a program will cost more than $100 billion dollars.
I will not attempt to estimate the additional cost associated with launching Mars rockets from the moon.
3.2 Risk. There are both operational and programmatic risks with any lunar program.
I believe the risk of going back to the moon is not substantially less than the risk of going directly to Mars. The major safety advantage of operations on the moon versus operations on Mars is the ability to recover people to the earth in a few days. This can be important in some situations, but it does not reduce the risks associated with launch, landing, or long duration operations in vacuum. And our prior experience and knowledge of lunar operations does not protect against errors in manufacturing quality control, human errors in preflight processing and real-time decisions, and unforeseen events and conditions.
The programmatic risk is even greater in my opinion. My logic goes like this:
Unmitigated success will be expected by the government and the public; after all we did this more than thirty years ago, so it should be no big deal to do it again. On the other hand, loss of a vehicle or crew will be trumpeted by critics of the program as clear evidence that we are not capable of going to Mars (e.g., “They can’t even do the things they did 30 years ago!”)
Furthermore, the lunar “stepping stone” portion of the Mars program will not reduce total HMEP costs. In fact it may become a very expensive sidetrack that competes with the funding needed to carry out the work to initiate Mars flights.
Lastly, our sole focus on the “Mars initiative” will make us incapable of dealing with any other human flight opportunity (maybe an unexpected chance to view some fantastic event if only Hubble were in good shape, or an unexpected science breakthrough on the ISS).
I’m just not convinced that returning to the moon is the best way to proceed to Mars.