High Tide on Europa
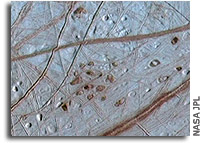
Jupiter’s large moons, called the Galilean satellites are heated by their orbits, literally.
The effect is known as tidal flexing, which arises from their resonant orbits, provides heat for volcanism on Io and could result in the presence of liquid water beneath Europa’s icy surface. There is good evidence for liquid water on Europa based on geological evidence from images of Europa taken by the Voyager and Galileo spacecraft. This geological evidence is tantalizing, but incomplete – it suggests that liquid water could be present, but also allows for the possibility that the strange features we see on Europa’s surface could all have formed through the motion of soft ice, without any liquid water at all.
The icy cracks of Jupiter’s moon Europa continue to intrigue astrobiologists. The white sheen is likely frost and the moon’s heat source is a combination of an underground ocean and tidal heating under the strong gravitational pull of Jupiter. Credit: Galileo Project, JPL, NASA
Fortunately, there are other methods available, in addition to geological techniques, which can provide information about the presence, or absence, of water at Europa. Thermal models of Europa’s subsurface are one theoretical way to study what lies beneath Europa’s surface, and we will consider them in this article.
Models of Europa’s gravitational field show that Europa possesses a surface layer about 100 km thick of material with the density of water, on top of a rocky interior and a metallic core. The surface layer is most likely H2O, but since the densities of solid ice and liquid water are very close, gravity models cannot distinguish between the two. So we know that there is about 100 km of some combination of water and/or ice at Europa’s surface, but other than knowing that the very top of this water layer is frozen solid, we do not know how thick the surface ice layer is, or if there is liquid water under it at some depth.
Thermal models of Europa’s subsurface suggest that it is possible, but not definite, that liquid water could be present. Thermal models include sources of heat and methods of cooling, and attempt to determine the thermal gradient and state (solid or liquid) of subsurface materials.
In the case of Europa, these models include heating from tidal dissipation and radiogenic sources, and cooling due to conduction and convection of heat. Tidal heating, as discussed in a previous article comes from the flexing of Europa by Jupiter’s gravity, as a result of Europa’s non-circular orbit due to its resonance with Io and Ganymede. As Europa’s distance from Jupiter changes over the course of its orbit, Jupiter’s gravitational attraction changes (since gravitational force is dependent on distance), and thus the tidal bulge raised by Jupiter goes up and down. This flexing causes heating of Europa’s subsurface.
Radiogenic heating is caused by the decay of long-lived radioactive isotopes that were incorporated in Europa when it formed, or brought to it after formation. Conduction is the direct transfer of heat from warmer to cooler regions, while convection is heat transfer due to motion of the materials – warmer materials move upwards, and cooler materials move downwards. Both conduction and convection result in the transfer of heat from Europa’s warmer interior to its frigid surface, and the net cooling of Europa as a result.
For Europa, thermal models that include all of these effects have been inconclusive. Some models have predicted that convection would remove all the heat from a liquid layer, resulting in Europa being frozen solid rather quickly. Other models have predicted that it would be difficult to produce enough heat to melt a solid ice layer into water, but that if a water layer existed there would be enough heat to maintain it as liquid indefinitely, due to a balance of cooling and heating sources.
There are still a number of unknown quantities in these models. For example, tidal heating is the most important heat source at Europa, but also the most poorly known. It is strongly affected by the rheology of ice, which is its behavior when pushed or pulled or squeezed. However, the rheology of ice is difficult to study under conditions similar to Europa, since Europa’s surface temperature is a chilly 100 K! It is also hard to study ice being stretched over the long periods associated with Europa’s tidal cycle – laboratory measurements are easier to make over time periods of seconds, not days.
We also don’t know enough about the composition of the ice on Europa – most models assume that it is pure water ice, but a small amount of other material present in the ice, especially other volatiles such as ammonia or salts, could dramatically alter the rheology of Europa’s ice. Most models also assume that the ice layer is solid, but the rheology could be changed if the ice layer is broken or fractured, or if the grain size is different than assumed in the models.
So in addition to the geological evidence, thermal models provide one more tantalizing, yet insufficient, clue to Europa’s subsurface structure. Water could be present, but is not required. Fortunately, however, we have some more definite evidence for subsurface water on Europa. It comes from an unlikely source – magnetic field measurements.
Timely Ends
The Galileo spacecraft, which officially finishes its mission this week in the Jovian system, took a number of images of Europa’s surface between 1995 and 2001 that can be used to study the geology of this small moon and look for evidence of liquid water beneath the surface.
The Voyager spacecraft took the first close-up images of Europa in the late 1970’s. Before Voyager, little was known about Europa’s surface except that it was very bright, and measurements taken from Earth with spectrometers on telescopes suggested that there could be water. Images of Europa taken by Voyager revealed a surface covered with crack-like features, and very few impact craters. The lack of craters was surprising, since all bodies in the solar system are continually hit by debris. This process results in a pockmarked surface like the Moon’s unless geologic activity takes place to remove craters from the surface. The relative lack of craters on Europa means that the surface is young, perhaps as young as a few tens of million years.
Images of Europa’s surface taken by the Galileo spacecraft have shown surface features that could be consistent with the presence of liquid water beneath Europa’s surface, but do not prove it. Europa’s surface is primarily covered by a vast set of interconnecting cracks and ridges. Also present are areas of disrupted “chaotic terrain”, where the surface appears to have been broken up into coherent iceberg-like blocks that seem to have “rafted” into new positions. Such areas can be reconstructed by fitting the preexisting features on the blocks back together like pieces of a jigsaw puzzle. Other features of interest on Europa’s surface include regions that could possibly be low viscosity surface flows, and impact craters that are anomalously shallow.
A number of models have been proposed for the formation of the variety of features visible in Galileo images of Europa’s surface. There is currently no consensus among these often-contradictory views of Europa’s geophysics. In general, models of Europa’s subsurface fall into two categories, one in which a thin ice layer (at most a few kilometers thick) is present on top of a layer of liquid water, and another in which the surface ice layer is much thicker, perhaps tens of kilometers or more, with liquid water (if it exists) at a much lower depth. Models of the formation of various geologic features on Europa seem to follow either the thin-ice or the thick-ice view.
For example, the shapes of Europa’s impact craters suggests that they formed within a solid target, but their shallow depths suggest that the surface rebounded somewhat after their formation. Models of this rebound suggest that most craters on Europa formed in a 5-15 km thick brittle surface layer, overlying a lower-viscosity subsurface layer. This subsurface material, however, could either be liquid water or warm, low-viscosity ice. A separate model of central peak formation in Europan craters suggests that the ice crust layer must be at least 3-4 km thick, and a model of crater topography suggests a much thicker ice layer, at least 19-25 km.
Cryovolcanic surface flows, made of a mixture of water, ice, and maybe other volatile materials such as ammonia, would be intriguing, but there are very few regions where the shape of surface features is suggestive of flows. There is also a substantial buoyancy problem in their formation, as it is difficult to get liquid water to the surface of Europa since it is denser than ice. One possible such region is visible in the figure, and is about 3 km across. This region could have been formed when some type of fluid-like material covered over pre-existing ridges and other features.
Models of ridge formation range from cryovolcanism to tidal squeezing to linear diapirs to compression and plastic deformation. These models range in requirements from a very thin crust overlying liquid water (the tidal squeezing model) to completely solid-state models with a thin brittle crust on top of a lower-viscosity, warm ice layer (diapirism or compression). One interesting feature called cycloidal ridges seem to correspond in orientation and location to cracking of the surface in response to the changing daily tidal stresses. This model would require the existence of a global ocean near the surface to obtain sufficient tidal stresses to crack the ice. Clearly, current models in the literature are contradictory and have very different implications for Europa’s subsurface structure.
Chaotic terrain was first seen as a “smoking gun” for the presence of liquid water beneath Europa’s surface, but formation models that involve only solid materials are also possible. At the liquid end of the spectrum, regions of chaotic terrain are seen as areas of localized heat flow where the ice layer melted all the way to the surface. In this model, the blocks are buoyant remnants of the preexisting icy crust that move about in a slushy matrix, both translating and tilting. Eventually the matrix freezes solid, ending the blocks’ motion and preserving their final positions. This model requires localized heating of the crust, but it may be difficult to concentrate the heating in both space and time. The solid-state formation model suggests that ice rises to the surface in a diapir, eventually disrupting the brittle surface. These convective upwellings make it difficult to tilt the blocks as observed, however. A third, intermediate model suggests that runaway melting within rising diapers produces chaos. Thus, models of chaos formation do seem to favor the existence of either water or an ice-water slurry at shallow depths near the surface, but such patches could be localized and not require the existence of a global liquid ocean layer.
Europa Will Never be Done
Although the Galileo satellite is scheduled to dive into Jupiter’s atmosphere on September 21, 2003, Phillips states with confidence that “Europa will never be done,” and adds, “I could happily work on Europa for another 20 years.”
For the next five of those years, she’ll be using the Galileo data set, but she acknowledges that this data has limitations. The failure of Galileo’s high gain antenna limited the quality of the images. Also, the satellite spent a relatively limited amount of time observing Europa as it orbited Jupiter, imaging the planet and its other moons in addition to the object of her study.
The quality of the combined images of Galileo and Voyager is frustratingly inconsistent. “Some areas,” she says, “have a resolution of 20 kilometers per pixel some are very sharp, some are very fuzzy.” While scientists do have images of the entire surface, some are, in Phillips’ words, “pretty lousy.” In some areas of Europa, “Voyager 2 images are better than Galileo– we’re using these.”
Phillips worked with the U.S. Geological Survey building a map of Europa, “trying to blend a hodge-podge of images into smoothest map possible.” Looking at a globe of the moon, it is possible to see large areas where the features are still sketchy. “There are regions where we just need better data.”
She eagerly anticipates the next NASA mission to the mysterious planet, tentatively planned as the “Jupiter Icy Moons Orbiter” or JIMO mission, which may launch in “about a decade” and would orbit Europa for up to a month. “JIMO would take a very nice consistent data set of the kind I’ve been hoping for,” she explains. “I’m crossing my fingers.”